Abstract
Rationale
Overeating beyond individuals' homeostatic needs critically contributes to obesity. The neurobehavioral mechanisms underlying the motivation to consume excessive foods with high calories are not fully understood.Objective
The present study examined whether a pharmacological stressor, yohimbine, enhances the motivation to procure food reward with an emphasis on comparisons between standard lab chow and high-fat foods. The effects of corticotropin-releasing factor (CRF) receptor blockade by a CRF1-selective antagonist NBI on the stress-enhanced motivation for food reward were also assessed.Methods
Male Sprague-Dawley rats with chow available ad libitum in their home cages were trained to press a lever under a progressive ratio schedule for deliveries of either standard or high-fat food pellets. For testing yohimbine stress effects, rats received an intraperitoneal administration of yohimbine 10 min before start of the test sessions. For testing effects of CRF1 receptor blockade on stress responses, NBI was administered 20 min prior to yohimbine challenge.Results
The rats emitted higher levels of lever responses to procure the high-fat food pellets compared with their counterparts on standard food pellets. Yohimbine challenge facilitated lever responses for the reward in all of the rats, whereas the effect was more robust in the rats on high-fat food pellets compared with their counterparts on standard food pellets. An inhibitory effect of pretreatment with NBI was observed on the enhancing effect of yohimbine challenge but not on the responses under baseline condition without yohimbine administration.Conclusions
Stress challenge significantly enhanced the motivation of satiated rats to procure extra food reward, especially the high-fat food pellets. Activation of CRF1 receptors is required for the stress-enhanced motivation for food reward. These results may have implications for our better understanding of the biobehavioral mechanisms of overeating and obesity.Free full text

Enhanced motivation for food reward induced by stress and attenuation by corticotrophin-releasing factor receptor antagonism in rats: implications for overeating and obesity
Abstract
Rationale
Overeating beyond individuals’ homeostatic needs critically contributes to obesity. The neurobehavioral mechanisms underlying the motivation to consume excessive foods with high calories are not fully understood.
Objective
The present study examined whether a pharmacological stressor, yohimbine enhances the motivation to procure food reward with an emphasis on comparisons between standard lab chow and high-fat foods. The effects of corticotropin-releasing factor receptor (CRF1) blockade by a CFR1 selective antagonist NBI on the stress-enhanced motivation for food reward were also assessed.
Methods
Male Sprague-Dawley rats with chow available ad libitum in their home cages were trained to press a lever under a progressive-ratio schedule for deliveries of either standard or high-fat food pellets. For testing yohimbine stress effects, rats received an intraperitoneal administration of yohimbine 10 min before start of the test sessions. For testing effects of CRF1 receptor blockade on stress responses, NBI was administered 20 min prior to yohimbine challenge.
Results
The rats emitted higher levels of lever responses to procure the high-fat food pellets compared with their counterparts on standard food pellets. Yohimbine challenge facilitated lever responses for the reward in all of the rats, whereas the effect was more robust in the rats on high-fat food pellets compared with their counterparts on standard food pellets. An inhibitory effect of pretreatment with NBI was observed on the enhancing effect of yohimbine challenge but not on the responses under baseline condition without yohimbine administration.
Conclusions
Stress challenge significantly enhanced the motivation of satiated rats to procure extra food reward, especially the high-fat food pellets. Activation of CRF1 receptors is required for the stress-enhanced motivation for food reward. These results may have implications for our better understanding of the biobehavioral mechanisms of overeating and obesity.
Introduction
Obesity has been an ever-increasing health problem in developed countries over the past three decades. In the United States, approximately two thirds of the population can be regarded as overweight, with a body mass index ≥ 25 kg/m2 (Flegal et al., 2010). In the classic view, the human body regulates feeding behavior homeostatically. Hormonal regulators, such as insulin, leptin, neuropeptide Y, ghrelin, and others, act on specific brain regions, mainly the hypothalamus and hindbrain, to maintain homeostatic food intake and energy balance (Abizaid et al., 2006; Berthoud, 2012; Gao and Horvath, 2007; Havel, 2001; Steffens and Strubbe, 1987; Sumithran and Proietto, 2013; Williams and Elmquist, 2012; Woods et al., 1998). Disturbances in the homeostasis of the system that governs food intake result in an imbalance between the accumulation and expenditure of energy, leading to pathophysiological states. For example, the dysfunction of hormonal regulators, such as leptin deficiency, leads to overeating and positive energy balance, resulting in the development of obesity (Duca and Covasa; Gautron and Elmquist, 2011; Herzog, 2003). However, given the extreme rarity of genetic causes of obesity (Ramachandrappa and Farooqi, 2011), unlikelihood that human genetic changes have substantially accrued over the past three decades, and fact that energy expenditure through physical activities has not dramatically decreased from 1980 to 2005 (Westerterp and Speakman, 2008), a reasonable proposition is that the exponential increase in the prevalence of obesity may be attributable to environmental and psychological factors that are present in our ever-changing societies (Berthoud, 2012; Berthoud and Morrison, 2008).
Stress exerts significant effects on both the energy homeostasis system and the brain regions involved in reward processing such as the mesocorticolimbic dopamine circuit, resulting in changes in feeding behavior and food intake. Human studies have suggested a close relationship between altered eating behavior, obesity, with stress in life (Adam and Epel, 2007; Block et al., 2009; Brunner et al., 2007; Dallman, 2010; Dallman et al., 2005; Kyrou et al., 2006; Lemmens et al., 2011; Maniam and Morris, 2012; Nieuwenhuizen and Rutters, 2008; Sinha and Jastreboff, 2013; Torres and Nowson, 2007). Although people eat less under conditions of extreme and life-threatening stress as a component of “flight or fight” responses, exposure to moderately stressful situations that are increasingly encountered in modern society increases food intake and especially the consumption of foods that have a high content of sugar and fat, even in cases in which the individuals are not hungry (Berthoud, 2004; Born et al., 2010; Epel et al., 2001; Lemmens et al., 2011; Oliver et al., 2000; Rutters et al., 2009; Zellner et al., 2006). Conversely, reports show that the successful maintenance of weight loss is associated with a lower level of stress and better ability to cope with stress (Elfhag and Rossner, 2005; Sarlio-Lahteenkorva et al., 2000). Altogether, results from population-based studies and clinical laboratory studies indicate a significant role for stressful life events in the increasing prevalence of overeating and obesity in developed countries over the past three decades (Berghofer et al., 2008; Caballero, 2007; Flegal et al., 2010).
In animal studies, however, the effects of exposure to stressors on food intake have been inconsistent and depend on the accessibility to food and types of food provided. For example, stress-challenged rodents consumed less ordinary foods (Calvez et al., 2011; Dal-Zotto et al., 2004; Harris et al., 1998; Marti et al., 1994; Rybkin et al., 1997; Solomon et al., 2007; Valles et al., 2000) and exhibited an increase in the intake of energy-dense and palatable foods (Foster et al., 2009; Kinzig et al., 2008; la Fleur et al., 2005). These animal studies were performed using procedures that allowed the subjects to have ad libitum access to food in their home cages. Interestingly, in recent studies that used operant conditioning paradigms, stress consistently reinstated food-seeking responses without food availability during the test sessions in rats with a history of self-administering high-fat foods (Calu et al., 2013; Cifani et al., 2012; Ghitza et al., 2006; Ghitza et al., 2007; Le et al., 2011; Nair et al., 2009; Nair et al., 2008; Nair et al., 2006; Nair et al., 2011; Pickens et al., 2012; Richards et al., 2008). Based on this line of research, stress exposure is hypothesized to increase animals’ motivation for procuring food rewards, especially foods that have high energy contents.
To test this hypothesis, the present study examined the effects of a stress challenge on lever-pressing for food rewards. Several features were embedded in the experimental design. First, a progressive-ratio (PR) schedule of reinforcement was used to measure the motivation for the delivery of rewards (Markou et al., 1993). The PR schedule provided information on the effort that the rats were willingly to expend to earn the delivery of food pellets. Second, an emphasis was placed on comparisons between standard lab chow and high-fat food reward. Third, the animals were satiated in terms of food consumption and energy balance because they had ad libitum access to standard lab chow in their home cages. This allowed examination of the animals’ motivation for gaining excessive food rewards beyond the need to maintain energy homeostasis. Fourth, a pharmacological stressor, yohimbine, was administered before the test sessions. Yohimbine is an α2 adrenergic receptor antagonist that increases the activity of noradrenergic systems, including the neural structures implicated in stress responses (Abercrombie et al., 1988; Aghajanian and VanderMaelen, 1982; Chopin et al., 1986; Uhde et al., 1984). Yohimbine also produces anxiety- and stress-like states in humans and laboratory animals (Bremner et al., 1996a; b; Charney et al., 1983; Davis et al., 1979; Holmberg and Gershon, 1961; Lang and Gershon, 1963). Therefore, yohimbine has been increasingly used as a stressor in experimental studies, especially in the field of drug addiction research, including our own work (Cippitelli et al., 2010; Feltenstein and See, 2006; Funk et al., 2006; Ghitza et al., 2006; Kupferschmidt et al., 2009; Le et al., 2005; Lee et al., 2004; Liu, 2010; 2012; Nair et al., 2006; Schroeder et al., 2003; Shepard et al., 2004; Zarrindast et al., 2000). Importantly, the behavioral effects of yohimbine-induced stress appear to be more robust than the stress elicited by classically used footshock (Bossert et al., 2005; Le and Shaham, 2002; Lu et al., 2003; Shaham et al., 2000).
In using yohimbine as a pharmacological stressor, it should be acknowledged that central noradrenergic neurotransmission has long been known to participate in the regulation of feeding behavior and food intake (Grossman, 1960; Leibowitz, 1986; Wellman, 2005). Interestingly, however, the role of norepinephrine in food intake seems to be inconclusive and mounting literature has documented norepinephrine to both stimulate and suppress eating behavior, which might be attributable to the activation of distinct adrenoceptor subtypes (Bello et al., 2013; dos Santos et al., 2009; Jackson et al., 1997; Le et al., 2011; Swiergiel and Wieczorek, 2008; Zislis et al., 2007). Moreover, the corticotropin-releasing factor (CRF) systems in both the hypothalamic-pituitary-adrenal (HPA) axis and extrahypothalamic regions such as the extended amygdala circuits participate in the automomic and emotional and behavioral responses to stresses. This study further examined involvement of CRF in mediating yohimbine effects on motivation for food reward by blockade of CRF receptors (CRF1). Specifically, a CRF1 antagonist NBI (5-chloro-N-[cyclopropylmethyl]-2-methyl-N-propyl-N’-[2,4,6-trichlorophenyl]-4,6-pyrimidinediamine hydrochloride) was administered prior to yohimbine challenge in rats that responded under the PR schedule for deliveries of either standard or high-fat food pellets.
Materials and methods
Animals
Male Sprague-Dawley rats (Charles River, Portage, MI), 201-225 g upon arrival, were used. The animals were individually housed in a humidity- and temperature-controlled (21-22°C) colony room maintained on a 12 h/12 h reverse light/dark cycle (lights off at 8:00 AM). During the first week after arrival, the rats were allowed to acclimate to the new environment and daily handling. The rats had unlimited access to water and laboratory rodent chow (Harlan rodent diet, 22% protein, 5.5% fat, 40.6% carbohydrate and 3.9% fiber), with the exception of a brief period of food restriction during lever-press training as described below. The experimental sessions were conducted during the dark phase at the same time each day (9:00 AM-3:00 PM). All of the experimental procedures were performed in accordance with the National Institutes of Health Guide for the Care and Use of Laboratory Animals and approved by the University of Mississippi Medical Center Institutional Animal Care and Use Committee.
Experimental apparatus
Standard operant conditioning chambers located inside sound-attenuating, ventilated cubicles (Med Associates, St. Albans, VT) were used. The chambers were equipped with two retractable response levers on one side panel, a 28-V white light above each lever, and a red house light on the upper center of the opposite panel. Between the two levers was a food pellet trough. Experimental events and data collection were automatically controlled by a computer and software (Med Associates, Med-PC version IV).
Lever-press training
After 1 week of acclimation, the rats were placed on a food restriction regimen with 20 g chow/day to facilitate the learning of operant responding for food reward. In the daily training sessions, the rats were placed in the experimental chambers, and the sessions began with the introduction of one lever. Responding on the lever was rewarded with the delivery of a food pellet (45 mg). The sessions lasted 1 h with a maximum delivery of 45 food pellets on a fixed-ratio 1 (FR1) schedule. After the rats learned to respond, the reinforcement schedule was increased to FR5. The training sessions finished once the rats earned 45 food pellets on the FR5 schedule in a single session. Successful lever-press training was achieved within 2-5 sessions. Thus, the food restriction regimen lasted at most 5 days. After the lever-press training and throughout the subsequent experiments, the rats had free access to chow in their home cages.
Food self-administration under a PR schedule
After lever-press training, the rats were subjected to daily 1-h food self-administration sessions. The experimental sessions began with introduction of the two levers. Responses on the active level were reinforced with the delivery of food pellets. To measure the rats’ motivation for obtaining food reward, a PR schedule of reinforcement was used. The PR schedule was modified from the formula 5e(0.2 × infusion number)−5 (Depoortere et al. 1993), which evolved from the original schedule requiring a fixed increment of lever presses for each consecutive reinforce delivery (Hodos, 1961). Thus, the response requirement for successive food pellet delivery was 3, 6, 10, 15, 20, 25, 32, 40, 50, 62, 77, 95, 118, 145, 179, 219, 268, and so on. Each food pellet delivery was signaled with the presentation of a stimulus that consisted of a 5 s tone and illumination of the light above the active lever for 20 s. A 20 s timeout period followed each delivery of the reinforcer, during which time the active lever responses resulted in no consequence but were recorded. Throughout the experiments, responses on the second, inactive lever did not produce any programmed consequence.
Standard food vs. high-fat food pellets
Separate groups of rats self-administered either standard food pellets or high-fat food pellets. The food pellets were purchased from Bio-Serv, an animal diet supplier (Frenchtown, NJ). Standard food pellets are nutritionally balanced with 21.3% protein, 3.8% fat, 54% carbohydrate and 4% fiber whereas high-fat food pellets contain 35% fatty content, 12.6% protein, 41.3% carbohydrate and 3.1% fiber. To some extent, the standard food pellets and high-fat food pellets had differences in taste and palatability, with the latter being more palatable for rats.
Yohimbine challenge tests
After establishing under the PR schedule stable self-administration of standard food pellets (n = 8) or high-fat food pellets (n = 8), effects of yohimbine were tested. Specifically, ten minutes before the test sessions, rats received intraperitoneal administration of yohimbine (0, 1, and 2.5 mg/kg) in a within-subjects Latin-square design. Thus, rats in either group were subjected to administration of each yohimbine dose only once in a pseudorandom manner. The test sessions were conducted under conditions that were exactly the same as described above for the self-administration sessions, during which responses on the active lever were reinforced by deliveries of either standard food pellets or high-fat food pellets. The yohimbine challenge tests were scheduled with two non-treatment sessions in between to eliminate any possible carry-over effects.
Effects of NBI on yohimbine-enhanced motivation for food reward
Ten rats were used to test the effects of the CRF1 receptor antagonist NBI on yohimbine-induced enhancement of the motivation for self-administration of high-fat food pellets. After establishment of stable responses for high-fat food pellets on the PR schedule, the test sessions were conducted. Thirty minutes before the tests, the rats were subcutaneously administered NBI (5-chloro-N-[cyclopropylmethyl]-2-methyl-N-propyl-N’-[2,4,6-trichlorophenyl]-4,6-pyrimidinediamine hydrochloride; Tocris Bioscience) at doses of 0, 5, and 10 mg/kg in a within-subjects Latin-square design. Twenty minutes later, all of the rats received an intraperitoneal injection of yohimbine at a dose of 2.5 mg/kg (which produced the most robust effects in the aforementioned yohimbine challenge tests). Ten minutes after yohimbine administration, the test sessions were performed and designed with two non-treatment sessions in between.
Rats (n = 10) trained with standard food pellets were subjected to the test procedure exactly as described above for their high-fat pellet counterparts. That is, rats received first NBI and then yohimbine administration prior to the test sessions.
To control for the possibility that NBI produced an effect on responding for food pellets independently of yohimbine challenge, rats trained with either standard or high-fat food pellets (n = 9 each) were tested for the effects of NBI on lever responses without yohimbine challenge. Specifically, rats were subjected to a subcutaneous administration of NBI at 10 mg/kg 30 min before the test session. Twenty minutes later, they got an intraperitoneal injection of saline rather than yohimbine. The test session was identical to that performed in the aforementioned training phase.
Data analysis
The data are expressed as the mean ± SEM number of lever responses, food pellets earned, and break points for active lever responses. A two-way repeated-measures analysis of variance (ANOVA) was used first to compare the two types of food pellets under both self-administration baseline and yohimbine challenge conditions. Then, following a significant interaction, the data obtained for the two food pellet types were analyzed separately. A one-way repeated-measures ANOVA was used to analyze the data obtained from drug treatment tests using a within-subjects Latin-square design. The Newman-Keuls post hoc test was used to verify differences among individual means.
Results
Food self-administration: comparison between standard food pellets and high-fat food pellets
The rats successfully learned to press a lever for food self-administration in the daily 1-h sessions under a PR schedule of reinforcement. As shown in Fig. 1a, the rats that worked for the delivery of high-fat food pellets emitted a greater number of lever-press responses compared with their counterparts that responded for standard food pellets. The two-way repeated-measures ANOVA showed significant effects of food pellet type (F1,14 = 5.15, p < 0.05) and session (F11,154 = 3.53, p < 0.001) and a significant pellet × session interaction (F11,154 = 2.32, p < 0.05). Rats that responded for high-fat food pellets exhibited a significantly higher break point for active lever responses (F1,14 = 5.19, p < 0.05; Fig. 1b) and earned more pellets (F1,14 = 4.67, p < 0.05; Fig. 1c) compared with the rats that responded for standard food pellets. In contrast, no difference was found in the number of responses on the inactive lever between the rats that self-administered standard vs. high-fat food pellets (data not shown). As shown in Fig. 1d, the rats in these two groups had similar levels of weight gain (F1,14 = 5.19, p > 0.05).
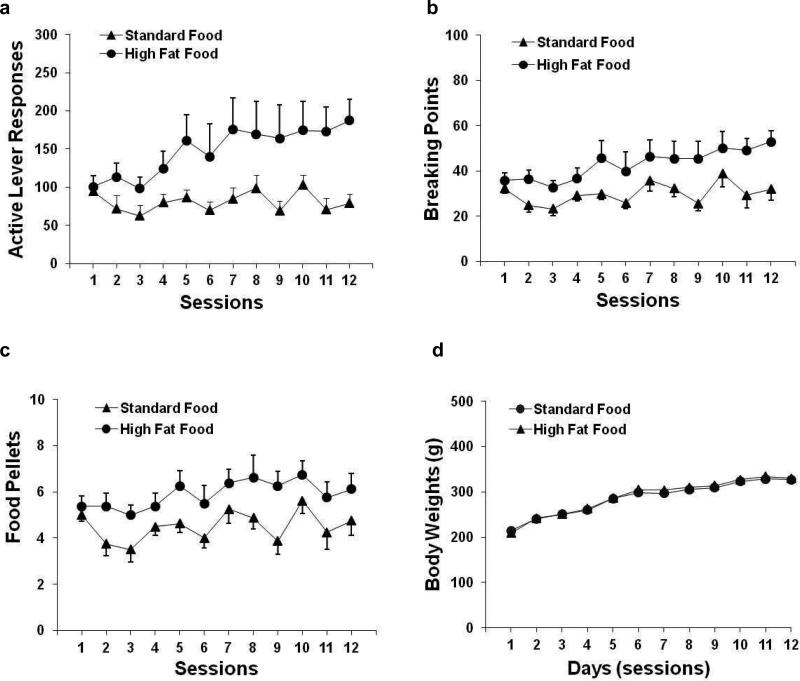
Profiles of active lever responses (a) and breaking points (b), food pellets earned (c), and body weights (d) in rats (n = 8 in each group) that had free access to standard laboratory chow in their home cages and received daily 1-h sessions for food self-administration under a PR schedule of reinforcement.
Effect of yohimbine on the motivation to self-administer food: comparison between standard food pellets and high-fat food pellets
As shown in Fig. 2.a, the pharmacological stressor yohimbine significantly increased responses on the active lever for food self-administration. The two-way repeated-measures ANOVA of active lever responses revealed significant effects of food pellet type (F1,14 = 4.73, p < 0.05) and yohimbine dose (F2,28 = 16.47, p < 0.0001) and a significant pellet × dose interaction (F2,128 = 4.53, p < 0.05). The one-way repeated-measures ANOVA and subsequent Newman-Keuls post hoc test revealed a significant difference (p < 0.01) in the number of active lever responses after pretreatment with 1 and 2.5 mg/kg yohimbine compared with saline controls in rats that self-administered standard food. For the rats that self-administered high-fat food pellets, this statistical analysis revealed a significant difference in the number of active lever responses after yohimbine pretreatment at doses of 1 mg/kg (p < 0.05) and 2.5 mg/kg (p < 0.01) compared with saline controls and a significant difference between the 2.5 and 1 mg/kg yohimbine doses (p < 0.05). Similar differences in the break points for active lever responses and number of pellets self-administered after pretreatment with yohimbine were observed (Fig. 2c, d). However, responses on the inactive lever remained unchanged (Fig. 2b), with no effect of food pellet type (F1,14 = 0.00, p > 0.05) or yohimbine dose (F2,28 = 0.19, p > 0.05) and no pellet × dose interaction (F2,128 = 1.52, p > 0.05).
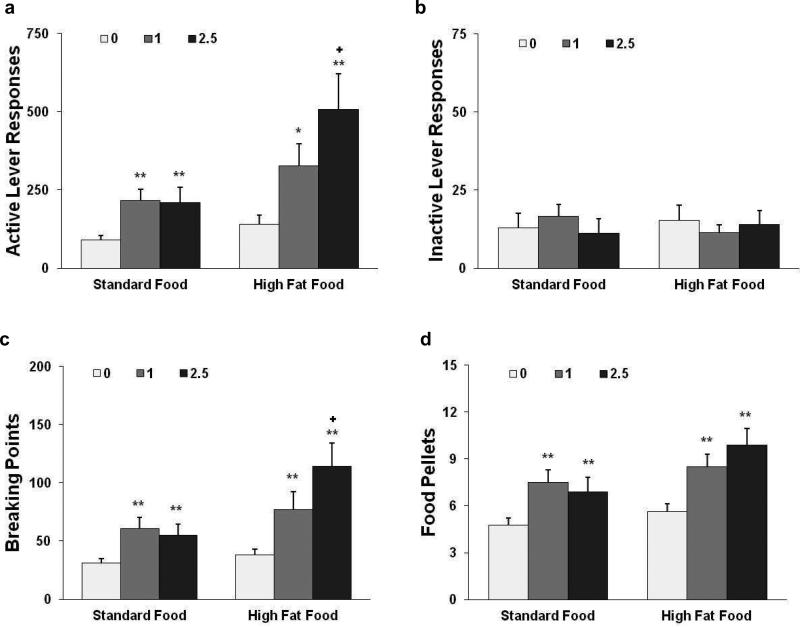
Effects of yohimbine challenge on active lever responses (a) and breaking points (c), food pellets earned (d), and inactive lever responses (b) in rats that under a PR schedule of reinforcement responded for deliveries of standard (n = 8) or high-fat (n=8) food pellets. The data are expressed as mean±SEM. *p < 0.05, **p < 0.01, significant difference from vehicle (0); +p < 0.05, significant difference from the low dose (1 mg/kg).
Effect of NBI on yohimbine-induced enhancement of the motivation for food reward
Pretreatment with the CRF1 antagonist NBI significantly reduced yohimbine-induced enhancement of the motivation to self-administer food pellets in the rats that had been trained with either the high-fat (Fig. 3a) or the standard (Fig. 3b) food pellets. In the animals on high-fat food pellets, a one-way repeated-measures ANOVA of the number of active lever responses revealed a significant effect of NBI dose (F2,18 = 14.06, p < 0.001). The subsequent Newman-Keuls post hoc test verified a significant difference (p < 0.01) between 10 mg/kg NBI treatment and both saline and 5 mg/kg NBI treatments. In the animals on standard food pellets, similar statistical analyses demonstrated a significant NBI dose effect (F2,18 = 5.13, p < 0.05) with a significant difference (p < 0.05) of 10 mg/kg NBI treatment versus saline control (Fig. 3b).

Effects of pretreatment with NBI on yohimbine-enhanced lever responses in rats that self-administered either high-fat (a) or standard (b) food pellets under a PR schedule of reinforcement. Panel c shows the number of responses on the active lever after NBI treatment without subsequent yohimbine challenge. The data are expressed as mean±SEM. *p < 0.05, **p < 0.01, significant difference from yohimbine+saline condition.
As shown in Fig. 3c, NBI pretreatment without subsequent yohimbine challenge did not change lever responses in the rats trained with either standard or high-fat food pellets.
In these drug treatment tests, however, responses on the inactive lever were unaltered.
Discussion
The present study examined the issue of whether stress alters rats’ motivation for food reward, with an emphasis on differences between the motivation for standard chow pellets vs. food pellets that have a high-fat content. The results showed three major findings. First, the food-satiated rats willingly emitted lever-press responses under a PR schedule of reinforcement for deliveries of food pellets. Moreover, the rats showed a significantly higher level of responding for the high-fat food pellets than for the standard chow pellets, indicating a stronger motivation for high-fat food even under food satiation conditions. Second, a pharmacological stress challenge, induced by administration of yohimbine, substantially enhanced lever-press responses for the delivery of food pellets. Particularly significant was the observation that the facilitating effect of stress was more robust in rats that responded for high-fat food pellets than in rats that responded for standard chow pellets. Third, pretreatment with the CRF1 receptor antagonist NBI attenuated the facilitating effect of yohimbine stress on the animals’ lever-press responses, indicating the involvement of CRF1 receptor activation in the stress-enhanced motivation for food reward.
Although numerous studies have investigated feeding behavior and weight changes within the framework of energy homeostasis, little is known about the psychological factors that contribute to overeating and obesity in subjects without a homeostatic need. Overeating and obese subjects procure and ingest foods beyond the need to maintain energy homeostasis. To closely simulate human overeating, the present study examined operant food self-administration in rats that were given free access to standard laboratory chow in their home cages; therefore, these animals were satiated in terms of food and energy intake. To properly interpret the lever-press response data, two speculations need to be addressed. First, in these rats, the high-fat food pellets might have caused general emotional arousal or elevated activity. The results showed that although the rats that responded for high-fat food pellets emitted significantly more responses on the active lever, they responded on the inactive lever at a very low level, similar to animals that responded for standard food pellets. Therefore, the rats emitted a higher number of lever-press responses specifically for deliveries of the high-fat food pellets rather than responded as a result of generally enhanced arousal or activity. Second, the significantly higher level of motivation for the high-fat pellets may have been attributable to the stimulation of multiple sensory receptors in the oral cavity by the lipid content of the high-fat pellets and not their high caloric value. Although this study did not directly address this issue, a recent study reported that mice readily emitted operant (lick) responses for direct intragastric infusions of lipid emulsions (Tellez et al., 2013), indicating that the stimulation of oral sensory receptors was not a critical factor for the self-administration of high-fat foods in rodents. Thus, oral sensations might have contributed little, if anything, to the self-administration responses observed in the present study.
The pharmacological stress induced by pre-session administration of yohimbine substantially enhanced lever-press responses for the delivery of food reward, regardless of the pellet type. The observation that yohimbine challenge did not change inactive lever responses ruled out the possibility of nonspecific activation of locomotor activity or operant behavior. These findings indicate that exposure to stress enhanced the rats’ motivation for earning food pellets, although these animals were satiated in terms of food intake and energy balance. With regard to understanding the facilitating effects of yohimbine on lever responses, each delivery of food pellets was associated with the presentation of auditory/visual stimuli that consisted of a 5 s tone and illumination of a light above the active lever for 20 s. Because of the repeated association with food reward, the stimuli acquired motivational value and became food-conditioned cues. Yohimbine challenge may also enhance the conditioned incentive effects of the food cue, and such an effect may have at least partially contributed to the increase in lever responses. Such a possibility is supported by previous studies. Shaham and colleagues used response-reinstatement procedures that have been widely employed in research on the reinstatement of drug-seeking behavior (Bossert et al., 2013; Epstein et al., 2006; Shaham et al., 2003). They demonstrated that acute stress induced by yohimbine reliably elicited operant food-seeking responses. The test sessions had response-contingent presentations of food-associated cues without the availability of food rewards (Calu et al., 2013; Cifani et al., 2012; Ghitza et al., 2006; Le et al., 2011; Nair et al., 2009; Nair et al., 2008; Nair et al., 2006; Nair et al., 2011; Pickens et al., 2012).
Notably, the yohimbine-induced enhancement of lever responses was significantly stronger in the rats that responded for high-fat food pellets compared with their counterparts that responded for standard pellets. These findings are consistent with observations in human subjects. For example, under controlled laboratory conditions, acute physical or emotional distress caused subjects to consume food with high-fat and sugar content, even when these individuals were not hungry and had no homeostatic need for calories (Epel et al., 2001; Foster et al., 2009; Lemmens et al., 2011; Oliver et al., 2000; Rutters et al., 2009). Overeating behavior is dissociable from individual physiological states of hunger and satiety but attributable to a substantial degree to exposure to environmental stimuli, such as stressful life events (Carels et al., 2004; Carels et al., 2001; Nishitani and Sakakibara, 2006; Nishitani et al., 2009; Oliver et al., 2001; Ozier et al., 2008; Schachter, 1968; Scott and Johnstone, 2012; Torres and Nowson, 2007). This lends support to earlier work suggesting that stress increased the consumption of and preference for nutrient-dense foods, particularly foods that have high-fat and sugar content (Kaplan and Kaplan, 1957). External and internal stimuli, such as stressful life events and changes in emotional states (either positive or negative), enhance the motivation for high-energy foods, overriding the basic homeostatic control of energy balance (Figlewicz, 2003; Gibson, 2006; Kelley et al., 2005; Zheng et al., 2009). In fact, the increase in stressful experiences in individuals in modern society has been proposed to promote eating foods that have high-fat and sugar content (Lattimore and Maxwell, 2004; O'Connor et al., 2008; Wallis and Hetherington, 2009).
Given that central noradrenergic neurotransmission is involved in the regulation of feeding behavior and food intake (Grossman, 1960; Leibowitz, 1986; Wellman, 2005) and that yohimbine via blocking presynaptic noradrenergic autoreceptors leads to activation of the noradrenergic system, it is reasonable to speculate that the enhancing effect of yohimbine on food reinforced responding might be a result of directly targeting the noradrenergic regulation of feeding behavior rather than a consequence of its interactions with stress-specific mechanisms. However, rat food intake in their home cages within 5 hours (yohimbine half-life is 7-8 hours) after yohimbine test sessions remained unchanged at a group level (data not shown). There was no consistent trend of either increase or decrease in food intake. Majority of animals in the yohimbine treated groups ate chow similar to saline vehicle rats with a couple rats consuming a little more while other a couple a little less chow. This observation is in line with literature showing the inconsistent effect of noradrenergic manipulations on food intake (Bello et al., 2013; dos Santos et al., 2009; Jackson et al., 1997; Le et al., 2011; Swiergiel and Wieczorek, 2008; Zislis et al., 2007). In operant procedures similar to that used in this study, neither activation nor blockade of postsynaptic noradrenergic receptors interfered with lever responses for the reinforcement with food pellets (Le et al., 2011) while a α2-adrenergic receptor agonist clonidine was found slightly decreased food pellet-supported lever responses (Zislis et al., 2007). Taken together, it is suggested that the facilitating effect of yohimbine challenge on lever responses in the present study is attributable to a unique role of yohimbine serving as a pharmacological stressor and thereby the stress-specific mechanisms for enhancing motivation (see below).
Previous animal research on eating behavior and weight changes was typically performed using home cage free-feeding procedures. Studies that use these types of eating paradigms to examine the effects of stress exposure on eating and weight gain have yielded inconsistent results. For example, some studies reported the inhibition of food intake and lower weight gain after exposure to stress (Bhatnagar et al., 2006; Calvez et al., 2011; Diane et al., 2008; Harris et al., 1998; Levin et al., 2000; Pecoraro et al., 2004; Ricart-Jane et al., 2002; Rybkin et al., 1997), which might be related to the effects of glucocorticoids, such as mobilizing energy stores and increasing hepatic gluconeogenesis (Dallman et al., 2005; Torres and Nowson, 2007). Other studies, in contrast, reported the overeating of foods, especially when foods with higher caloric value and palatability were provided, such as sweetened condensed milk (Bertiere et al., 1984; Marti et al., 1994; Miyasaka et al., 2005; Rowland and Antelman, 1976; Wallach et al., 1977). The procedures used in the present study, which measured operant responses for food rewards under a PR schedule of reinforcement and thereby examined the subjects’ motivation for procuring foods (Markou et al., 1993), and home-cage feeding paradigms appear to investigate different physiological processes that govern food intake and maintain energy balance. Such a distinction is supported by work that showed that although manipulations of mesocorticolimbic dopamine system function altered operant responses for food rewards, food intake in free-feeding regimens remained intact (Baldo and Kelley, 2007; Berridge, 2009; Salamone et al., 1994; Wirtshafter and Stratford, 2010).
In the present study, pretreatment with the CRF1 antagonist NBI prior to yohimbine challenge significantly attenuated lever responses in the rats that had been trained with either the standard or high-fat food pellets. That raises speculations that blockade of CRF1 receptors might directly reduce the lever responding for pellet reward rather than the yohimbine-enhanced motivation or that the suppressant effect of NBI might be due to the impairment of locomotor activity by the drug. However, such possibilities can be ruled out because NBI did not change lever responses in the tests where rats received NBI without a subsequent yohimbine stress challenge (a vehicle administration instead) and given that NBI at 10 mg/kg did not significantly alter food intake in 4 hours after administration (Giardino et al., 2013). Together, these data demonstrated that NIB pretreatment suppressed specifically the lever responses that were facilitated by yohimbine challenge rather than the pellet-supported responding itself. Moreover, such a suppressant effect of NIB on yohimbine-enhanced responding was observed regardless of the types of the food pellets that rats responded for. Because the levels of lever responses enhanced by yohimbine challenge were substantially different between the two types of pellets (208 ± 48 vs. 509 ± 118), the effect of NBI was independent of the levels of lever responses. Thus, the pharmacological blockade of CRF1 neurotransmission specifically reduced the yohimbine stress-enhanced motivation for food pellet reward.
The findings with NBI pretreatment in this study are in line with evidence generated in the drug addiction research field. For example, numerous studies have demonstrated the necessity of activation of CRF1 receptors in the expression of the stress-induced reinstatement of drug-seeking behavior (Bruijnzeel et al., 2009; Erb et al., 1998; Le et al., 2000; Liu and Weiss, 2002; Shaham et al., 1997; Zislis et al., 2007). More directly related to the present data is the finding that systemic antagonism of CRF1 receptors by antalarmin, a CRF1 antagonist, effectively suppressed the reinstatement of food-seeking responses induced by yohimbine (Ghitza et al., 2006). CRF is distributed in both the hypothalamus (as part of the HPA axis) and reward circuits, especially the extended amygdala, including the central nucleus of the amygdala, bed nucleus of the stria terminalis, and a transition area in the shell of the nucleus accumbens. Extrahypothalamic CRF plays an important role in behavioral responses to stress (Koob, 2009; Koob et al., 1993). Studies have demonstrated that exposure to stress elevated the level of CRF in the ventral tegmental area, and elevations of CRF facilitated dopamine neuronal activity, which was blocked by CRF1 antagonists (Rodaros et al., 2007; Wanat et al., 2008). Furthermore, extrahypothalamic CRF rather than HPA axis CRF plays a unique role in stress-induced reward-seeking behavior (Erb et al., 1998; Graf et al., 2011; Le et al., 2000). The present data, together with recent evidence of the overlap of neurobiological substrates for food- and drug-taking/seeking behavior (Kenny, 2011; Lutter and Nestler, 2009; Sinha and Jastreboff, 2013; Volkow et al., 2013 for example reviews), indicate that neurotransmission via the extrahypothalamic CRF system may play a role in heightened desire for food reward and overeating behavior in response to stressful life events, thereby contributing to the epidemic of obesity in an ever-modernizing society.
Acknowledgements
This work was supported by National Institutes of Health grant R01DA017288 and R01DA037277 from the National Institute on Drug Abuse and funds from the Department of Psychiatry and Human Behavior, University of Mississippi Medical Center. The author thanks Courtney Jernigan, Lisa Biswas, Laura Beloate, Ramachandram Avusula, Treniea Tolliver, Trisha Patel and Jonathan Lee for their excellent technical assistance.
References
- Abercrombie ED, Keller RW, Jr, Zigmond MJ. Characterization of hippocampal norepinephrine release as measured by microdialysis perfusion: pharmacological and behavioral studies. Neuroscience. 1988;27:897–904. [Abstract] [Google Scholar]
- Abizaid A, Gao Q, Horvath TL. Thoughts for food: brain mechanisms and peripheral energy balance. Neuron. 2006;51:691–702. [Abstract] [Google Scholar]
- Adam TC, Epel ES. Stress, eating and the reward system. Physiol Behav. 2007;91:449–458. [Abstract] [Google Scholar]
- Aghajanian GK, VanderMaelen CP. alpha 2-adrenoceptor-mediated hyperpolarization of locus coeruleus neurons: intracellular studies in vivo. Science. 1982;215:1394–1396. [Abstract] [Google Scholar]
- Baldo BA, Kelley AE. Discrete neurochemical coding of distinguishable motivational processes: insights from nucleus accumbens control of feeding. Psychopharmacology (Berl) 2007;191:439–459. [Abstract] [Google Scholar]
- Bello NT, Walters AL, Verpeut JL, Cunha PP. High-fat diet-induced alterations in the feeding suppression of low-dose nisoxetine, a selective norepinephrine reuptake inhibitor. J Obes. 2013;2013:457047. [Europe PMC free article] [Abstract] [Google Scholar]
- Berghofer A, Pischon T, Reinhold T, Apovian CM, Sharma AM, Willich SN. Obesity prevalence from a European perspective: a systematic review. BMC Public Health. 2008;8:200. [Europe PMC free article] [Abstract] [Google Scholar]
- Berridge KC. ‘Liking’ and ‘wanting’ food rewards: brain substrates and roles in eating disorders. Physiol Behav. 2009;97:537–550. [Europe PMC free article] [Abstract] [Google Scholar]
- Berthoud HR. Neural control of appetite: cross-talk between homeostatic and non-homeostatic systems. Appetite. 2004;43:315–317. [Abstract] [Google Scholar]
- Berthoud HR. The neurobiology of food intake in an obesogenic environment. Proc Nutr Soc. 2012;71:478–487. [Europe PMC free article] [Abstract] [Google Scholar]
- Berthoud HR, Morrison C. The brain, appetite, and obesity. Annu Rev Psychol. 2008;59:55–92. [Abstract] [Google Scholar]
- Bertiere MC, Sy TM, Baigts F, Mandenoff A, Apfelbaum M. Stress and sucrose hyperphagia: role of endogenous opiates. Pharmacol Biochem Behav. 1984;20:675–679. [Abstract] [Google Scholar]
- Bhatnagar S, Vining C, Iyer V, Kinni V. Changes in hypothalamic-pituitary-adrenal function, body temperature, body weight and food intake with repeated social stress exposure in rats. J Neuroendocrinol. 2006;18:13–24. [Abstract] [Google Scholar]
- Block JP, He Y, Zaslavsky AM, Ding L, Ayanian JZ. Psychosocial stress and change in weight among US adults. Am J Epidemiol. 2009;170:181–192. [Europe PMC free article] [Abstract] [Google Scholar]
- Born JM, Lemmens SG, Rutters F, Nieuwenhuizen AG, Formisano E, Goebel R, Westerterp-Plantenga MS. Acute stress and food-related reward activation in the brain during food choice during eating in the absence of hunger. Int J Obes (Lond) 2010;34:172–181. [Abstract] [Google Scholar]
- Bossert JM, Ghitza UE, Lu L, Epstein DH, Shaham Y. Neurobiology of relapse to heroin and cocaine seeking: an update and clinical implications. Eur J Pharmacol. 2005;526:36–50. [Abstract] [Google Scholar]
- Bossert JM, Marchant NJ, Calu DJ, Shaham Y. The reinstatement model of drug relapse: recent neurobiological findings, emerging research topics, and translational research. Psychopharmacology (Berl) 2013;229:453–476. [Europe PMC free article] [Abstract] [Google Scholar]
- Bremner JD, Krystal JH, Southwick SM, Charney DS. Noradrenergic mechanisms in stress and anxiety: I. Preclinical studies. Synapse. 1996a;23:28–38. [Abstract] [Google Scholar]
- Bremner JD, Krystal JH, Southwick SM, Charney DS. Noradrenergic mechanisms in stress and anxiety: II. Clinical studies. Synapse. 1996b;23:39–51. [Abstract] [Google Scholar]
- Bruijnzeel AW, Prado M, Isaac S. Corticotropin-releasing factor-1 receptor activation mediates nicotine withdrawal-induced deficit in brain reward function and stress-induced relapse. Biol Psychiatry. 2009;66:110–117. [Europe PMC free article] [Abstract] [Google Scholar]
- Brunner EJ, Chandola T, Marmot MG. Prospective effect of job strain on general and central obesity in the Whitehall II Study. Am J Epidemiol. 2007;165:828–837. [Abstract] [Google Scholar]
- Caballero B. The global epidemic of obesity: an overview. Epidemiol Rev. 2007;29:1–5. [Abstract] [Google Scholar]
- Calu DJ, Kawa AB, Marchant NJ, Navarre BM, Henderson MJ, Chen B, Yau HJ, Bossert JM, Schoenbaum G, Deisseroth K, Harvey BK, Hope BT, Shaham Y. Optogenetic inhibition of dorsal medial prefrontal cortex attenuates stress-induced reinstatement of palatable food seeking in female rats. J Neurosci. 2013;33:214–226. [Europe PMC free article] [Abstract] [Google Scholar]
- Calvez J, Fromentin G, Nadkarni N, Darcel N, Even P, Tome D, Ballet N, Chaumontet C. Inhibition of food intake induced by acute stress in rats is due to satiation effects. Physiol Behav. 2011;104:675–683. [Abstract] [Google Scholar]
- Carels RA, Douglass OM, Cacciapaglia HM, O'Brien WH. An ecological momentary assessment of relapse crises in dieting. J Consult Clin Psychol. 2004;72:341–348. [Abstract] [Google Scholar]
- Carels RA, Hoffman J, Collins A, Raber AC, Cacciapaglia H, O'Brien WH. Ecological momentary assessment of temptation and lapse in dieting. Eat Behav. 2001;2:307–321. [Abstract] [Google Scholar]
- Charney DS, Heninger GR, Redmond DE., Jr Yohimbine induced anxiety and increased noradrenergic function in humans: effects of diazepam and clonidine. Life Sci. 1983;33:19–29. [Abstract] [Google Scholar]
- Chopin P, Pellow S, File SE. The effects of yohimbine on exploratory and locomotor behaviour are attributable to its effects at noradrenaline and not at benzodiazepine receptors. Neuropharmacology. 1986;25:53–57. [Abstract] [Google Scholar]
- Cifani C, Koya E, Navarre BM, Calu DJ, Baumann MH, Marchant NJ, Liu QR, Khuc T, Pickel J, Lupica CR, Shaham Y, Hope BT. Medial prefrontal cortex neuronal activation and synaptic alterations after stress-induced reinstatement of palatable food seeking: a study using cfos-GFP transgenic female rats. J Neurosci. 2012;32:8480–8490. [Europe PMC free article] [Abstract] [Google Scholar]
- Cippitelli A, Damadzic R, Hansson AC, Singley E, Sommer WH, Eskay R, Thorsell A, Heilig M. Neuropeptide Y (NPY) suppresses yohimbine-induced reinstatement of alcohol seeking. Psychopharmacology (Berl) 2010;208:417–426. [Abstract] [Google Scholar]
- Dal-Zotto S, Marti O, Delgado R, Armario A. Potentiation of glucocorticoid release does not modify the long-term effects of a single exposure to immobilization stress. Psychopharmacology (Berl) 2004;177:230–237. [Abstract] [Google Scholar]
- Dallman MF. Stress-induced obesity and the emotional nervous system. Trends Endocrinol Metab. 2010;21:159–165. [Europe PMC free article] [Abstract] [Google Scholar]
- Dallman MF, Pecoraro NC, la Fleur SE. Chronic stress and comfort foods: self-medication and abdominal obesity. Brain Behav Immun. 2005;19:275–280. [Abstract] [Google Scholar]
- Davis M, Redmond DE, Jr, Baraban JM. Noradrenergic agonists and antagonists: effects on conditioned fear as measured by the potentiated startle paradigm. Psychopharmacology (Berl) 1979;65:111–118. [Abstract] [Google Scholar]
- Diane A, Victoriano M, Fromentin G, Tome D, Larue-Achagiotis C. Acute stress modifies food choice in Wistar male and female rats. Appetite. 2008;50:397–407. [Abstract] [Google Scholar]
- dos Santos RL, Mansur SS, Steffens SM, Faria MS, Marino-Neto J, Paschoalini MA. Food intake increased after injection of adrenaline into the median raphe nucleus of free-feeding rats. Behav Brain Res. 2009;197:411–416. [Abstract] [Google Scholar]
- Duca FA, Covasa M. Current and emerging concepts on the role of peripheral signals in the control of food intake and development of obesity. Br J Nutr. 108:778–793. [Abstract] [Google Scholar]
- Elfhag K, Rossner S. Who succeeds in maintaining weight loss? A conceptual review of factors associated with weight loss maintenance and weight regain. Obes Rev. 2005;6:67–85. [Abstract] [Google Scholar]
- Epel E, Lapidus R, McEwen B, Brownell K. Stress may add bite to appetite in women: a laboratory study of stress-induced cortisol and eating behavior. Psychoneuroendocrinology. 2001;26:37–49. [Abstract] [Google Scholar]
- Epstein DH, Preston KL, Stewart J, Shaham Y. Toward a model of drug relapse: an assessment of the validity of the reinstatement procedure. Psychopharmacology (Berl) 2006;189:1–16. [Europe PMC free article] [Abstract] [Google Scholar]
- Erb S, Shaham Y, Stewart J. The role of corticotropin-releasing factor and corticosterone in stress- and cocaine-induced relapse to cocaine seeking in rats. J Neurosci. 1998;18:5529–5536. [Europe PMC free article] [Abstract] [Google Scholar]
- Feltenstein MW, See RE. Potentiation of cue-induced reinstatement of cocaine-seeking in rats by the anxiogenic drug yohimbine. Behav Brain Res. 2006;174:1–8. [Abstract] [Google Scholar]
- Figlewicz DP. Adiposity signals and food reward: expanding the CNS roles of insulin and leptin. Am J Physiol Regul Integr Comp Physiol. 2003;284:R882–892. [Abstract] [Google Scholar]
- Flegal KM, Carroll MD, Ogden CL, Curtin LR. Prevalence and trends in obesity among US adults, 1999-2008. JAMA. 2010;303:235–241. [Abstract] [Google Scholar]
- Foster MT, Warne JP, Ginsberg AB, Horneman HF, Pecoraro NC, Akana SF, Dallman MF. Palatable foods, stress, and energy stores sculpt corticotropin-releasing factor, adrenocorticotropin, and corticosterone concentrations after restraint. Endocrinology. 2009;150:2325–2333. [Europe PMC free article] [Abstract] [Google Scholar]
- Funk D, Li Z, Le AD. Effects of environmental and pharmacological stressors on c-fos and corticotropin-releasing factor mRNA in rat brain: Relationship to the reinstatement of alcohol seeking. Neuroscience. 2006;138:235–243. [Abstract] [Google Scholar]
- Gao Q, Horvath TL. Neurobiology of feeding and energy expenditure. Annu Rev Neurosci. 2007;30:367–398. [Abstract] [Google Scholar]
- Gautron L, Elmquist JK. Sixteen years and counting: an update on leptin in energy balance. J Clin Invest. 2011;121:2087–2093. [Europe PMC free article] [Abstract] [Google Scholar]
- Ghitza UE, Gray SM, Epstein DH, Rice KC, Shaham Y. The anxiogenic drug yohimbine reinstates palatable food seeking in a rat relapse model: a role of CRF1 receptors. Neuropsychopharmacology. 2006;31:2188–2196. [Europe PMC free article] [Abstract] [Google Scholar]
- Ghitza UE, Nair SG, Golden SA, Gray SM, Uejima JL, Bossert JM, Shaham Y. Peptide YY3-36 decreases reinstatement of high-fat food seeking during dieting in a rat relapse model. J Neurosci. 2007;27:11522–11532. [Europe PMC free article] [Abstract] [Google Scholar]
- Giardino WJ, Ryabinin AE. CRF1 receptor signaling regulates food and fluid intake in the drinking-in-the-dark model of binge alchol consumption. Alcohol Clin Exp Res. 2013;37:1161–1170. [Europe PMC free article] [Abstract] [Google Scholar]
- Gibson EL. Emotional influences on food choice: sensory, physiological and psychological pathways. Physiol Behav. 2006;89:53–61. [Abstract] [Google Scholar]
- Graf EN, Hoks MA, Baumgardner J, Sierra J, Vranjkovic O, Bohr C, Baker DA, Mantsch JR. Adrenal activity during repeated long-access cocaine self-administration is required for later CRF-Induced and CRF-dependent stressor-induced reinstatement in rats. Neuropsychopharmacology. 2011;36:1444–1454. [Europe PMC free article] [Abstract] [Google Scholar]
- Grossman SP. Eating or drinking elicited by direct adrenergic or cholinergic stimulation of hypothalamus. Science. 1960;132:301–302. [Abstract] [Google Scholar]
- Harris RB, Zhou J, Youngblood BD, Rybkin, Smagin GN, Ryan DH. Effect of repeated stress on body weight and body composition of rats fed low- and high-fat diets. Am J Physiol. 1998;275:R1928–1938. [Abstract] [Google Scholar]
- Havel PJ. Peripheral signals conveying metabolic information to the brain: short-term and long-term regulation of food intake and energy homeostasis. Exp Biol Med (Maywood) 2001;226:963–977. [Abstract] [Google Scholar]
- Herzog H. Neuropeptide Y and energy homeostasis: insights from Y receptor knockout models. Eur J Pharmacol. 2003;480:21–29. [Abstract] [Google Scholar]
- Hodos W. Progressive ratio as a measure of reward strength. Science. 1961;134:943–944. [Abstract] [Google Scholar]
- Holmberg G, Gershon S. Autonomic and psychic effects of yohimbine hydrochloride. Psychopharmacologia. 1961;2:93–106. [Abstract] [Google Scholar]
- Jackson HC, Needham AM, Hutchins LJ, Mazurkiewicz SE, Heal DJ. Comparison of the effects of sibutramine and other monoamine reuptake inhibitors on food intake in the rat. Br J Pharmacol. 1997;121:1758–1762. [Europe PMC free article] [Abstract] [Google Scholar]
- Kaplan HI, Kaplan HS. The psychosomatic concepto of obesity. J Nerv Ment Dis. 1957;125:181–201. [Abstract] [Google Scholar]
- Kelley AE, Baldo BA, Pratt WE, Will MJ. Corticostriatal-hypothalamic circuitry and food motivation: integration of energy, action and reward. Physiol Behav. 2005;86:773–795. [Abstract] [Google Scholar]
- Kenny PJ. Reward mechanisms in obesity: new insights and future directions. Neuron. 2011;69:664–679. [Europe PMC free article] [Abstract] [Google Scholar]
- Kinzig KP, Hargrave SL, Honors MA. Binge-type eating attenuates corticosterone and hypophagic responses to restraint stress. Physiol Behav. 2008;95:108–113. [Abstract] [Google Scholar]
- Koob GF. Brain stress systems in the amygdala and addiction. Brain Res. 2009;1293:61–75. [Europe PMC free article] [Abstract] [Google Scholar]
- Koob GF, Heinrichs SC, Pich EM, Menzaghi F, Baldwin H, Miczek K, Britton KT. The role of corticotropin-releasing factor in behavioural responses to stress. Ciba Found Symp. 1993;172:277–289. discussion 290-275. [Abstract] [Google Scholar]
- Kupferschmidt DA, Tribe E, Erb S. Effects of repeated yohimbine on the extinction and reinstatement of cocaine seeking. Pharmacol Biochem Behav. 2009;91:473–480. [Abstract] [Google Scholar]
- Kyrou I, Chrousos GP, Tsigos C. Stress, visceral obesity, and metabolic complications. Ann N Y Acad Sci. 2006;1083:77–110. [Abstract] [Google Scholar]
- la Fleur SE, Houshyar H, Roy M, Dallman MF. Choice of lard, but not total lard calories, damps adrenocorticotropin responses to restraint. Endocrinology. 2005;146:2193–2199. [Abstract] [Google Scholar]
- Lang WJ, Gershon S. Effects of psychoactive drugs on yohimbine induced responses in conscious dogs. A proposed screening procedure for anti-anxiety agents. Arch Int Pharmacodyn Ther. 1963;142:457–472. [Abstract] [Google Scholar]
- Lattimore P, Maxwell L. Cognitive load, stress, and disinhibited eating. Eat Behav. 2004;5:315–324. [Abstract] [Google Scholar]
- Le A, Shaham Y. Neurobiology of relapse to alcohol in rats. Pharmacol Ther. 2002;94:137–156. [Abstract] [Google Scholar]
- Le AD, Funk D, Juzytsch W, Coen K, Navarre BM, Cifani C, Shaham Y. Effect of prazosin and guanfacine on stress-induced reinstatement of alcohol and food seeking in rats. Psychopharmacology (Berl) 2011;218:89–99. [Europe PMC free article] [Abstract] [Google Scholar]
- Le AD, Harding S, Juzytsch W, Funk D, Shaham Y. Role of alpha-2 adrenoceptors in stress-induced reinstatement of alcohol seeking and alcohol self-administration in rats. Psychopharmacology (Berl) 2005;179:366–373. [Abstract] [Google Scholar]
- Le AD, Harding S, Juzytsch W, Watchus J, Shalev U, Shaham Y. The role of corticotrophin-releasing factor in stress-induced relapse to alcohol-seeking behavior in rats. Psychopharmacology (Berl) 2000;150:317–324. [Abstract] [Google Scholar]
- Lee B, Tiefenbacher S, Platt DM, Spealman RD. Pharmacological blockade of alpha2-adrenoceptors induces reinstatement of cocaine-seeking behavior in squirrel monkeys. Neuropsychopharmacology. 2004;29:686–693. [Abstract] [Google Scholar]
- Leibowitz SF. Brain monoamines and peptides: role in the control of eating behavior. Federation proceedings. 1986;45:1396–1403. [Abstract] [Google Scholar]
- Lemmens SG, Rutters F, Born JM, Westerterp-Plantenga MS. Stress augments food ‘wanting’ and energy intake in visceral overweight subjects in the absence of hunger. Physiol Behav. 2011;103:157–163. [Abstract] [Google Scholar]
- Levin BE, Richard D, Michel C, Servatius R. Differential stress responsivity in diet-induced obese and resistant rats. Am J Physiol Regul Integr Comp Physiol. 2000;279:R1357–1364. [Abstract] [Google Scholar]
- Liu X. Contribution of drug cue, priming, and stress to reinstatement of nicotine-seeking behavior in a rat model of relapse. In: Egger J, KAlb M, editors. Smoking Relapse: Causes, Prevention, and Recovery. Nova Science Publisher; New York: 2010. pp. 143–163. [Google Scholar]
- Liu X. Stress as a risk factor for transition to heightened nicotine intake: evidence from a rat model of nicotine self-administration. In: Giovanni D, editor. Nicotine Addiction: Prevention, Health Effects and Treatment Options. Nova Science Publisher; Hauppauge, NY: 2012. pp. 115–134. [Google Scholar]
- Liu X, Weiss F. Additive effect of stress and drug cues on reinstatement of ethanol seeking: exacerbation by history of dependence and role of concurrent activation of corticotropin-releasing factor and opioid mechanisms. J Neurosci. 2002;22:7856–7861. [Europe PMC free article] [Abstract] [Google Scholar]
- Lu L, Shepard JD, Hall FS, Shaham Y. Effect of environmental stressors on opiate and psychostimulant reinforcement, reinstatement and discrimination in rats: a review. Neurosci Biobehav Rev. 2003;27:457–491. [Abstract] [Google Scholar]
- Lutter M, Nestler EJ. Homeostatic and hedonic signals interact in the regulation of food intake. J Nutr. 2009;139:629–632. [Europe PMC free article] [Abstract] [Google Scholar]
- Maniam J, Morris MJ. The link between stress and feeding behaviour. Neuropharmacology. 2012;63:97–110. [Abstract] [Google Scholar]
- Markou A, Weiss F, Gold LH, Caine SB, Schulteis G, Koob GF. Animal models of drug craving. Psychopharmacology (Berl) 1993;112:163–182. [Abstract] [Google Scholar]
- Marti O, Marti J, Armario A. Effects of chronic stress on food intake in rats: influence of stressor intensity and duration of daily exposure. Physiol Behav. 1994;55:747–753. [Abstract] [Google Scholar]
- Miyasaka K, Kanai S, Ohta M, Hosoya H, Takano S, Sekime A, Sakurai C, Kaneko T, Tahara S, Funakoshi A. Overeating after restraint stress in cholecystokinin-a receptor-deficient mice. Jpn J Physiol. 2005;55:285–291. [Abstract] [Google Scholar]
- Nair SG, Adams-Deutsch T, Pickens CL, Smith DG, Shaham Y. Effects of the MCH1 receptor antagonist SNAP 94847 on high-fat food-reinforced operant responding and reinstatement of food seeking in rats. Psychopharmacology (Berl) 2009;205:129–140. [Europe PMC free article] [Abstract] [Google Scholar]
- Nair SG, Golden SA, Shaham Y. Differential effects of the hypocretin 1 receptor antagonist SB 334867 on high-fat food self-administration and reinstatement of food seeking in rats. Br J Pharmacol. 2008;154:406–416. [Europe PMC free article] [Abstract] [Google Scholar]
- Nair SG, Gray SM, Ghitza UE. Role of food type in yohimbine- and pellet-priming-induced reinstatement of food seeking. Physiol Behav. 2006;88:559–566. [Europe PMC free article] [Abstract] [Google Scholar]
- Nair SG, Navarre BM, Cifani C, Pickens CL, Bossert JM, Shaham Y. Role of dorsal medial prefrontal cortex dopamine D1-family receptors in relapse to high-fat food seeking induced by the anxiogenic drug yohimbine. Neuropsychopharmacology. 2011;36:497–510. [Europe PMC free article] [Abstract] [Google Scholar]
- Nieuwenhuizen AG, Rutters F. The hypothalamic-pituitary-adrenal-axis in the regulation of energy balance. Physiol Behav. 2008;94:169–177. [Abstract] [Google Scholar]
- Nishitani N, Sakakibara H. Relationship of obesity to job stress and eating behavior in male Japanese workers. Int J Obes (Lond) 2006;30:528–533. [Abstract] [Google Scholar]
- Nishitani N, Sakakibara H, Akiyama I. Eating behavior related to obesity and job stress in male Japanese workers. Nutrition. 2009;25:45–50. [Abstract] [Google Scholar]
- O'Connor DB, Jones F, Conner M, McMillan B, Ferguson E. Effects of daily hassles and eating style on eating behavior. Health Psychol. 2008;27:S20–31. [Abstract] [Google Scholar]
- Oliver G, Wardle J, Gibson EL. Stress and food choice: a laboratory study. Psychosom Med. 2000;62:853–865. [Abstract] [Google Scholar]
- Oliver KG, Huon GF, Zadro L, Williams KD. The role of interpersonal stress in overeating among high and low disinhibitors. Eat Behav. 2001;2:19–26. [Abstract] [Google Scholar]
- Ozier AD, Kendrick OW, Leeper JD, Knol LL, Perko M, Burnham J. Overweight and obesity are associated with emotion- and stress-related eating as measured by the eating and appraisal due to emotions and stress questionnaire. J Am Diet Assoc. 2008;108:49–56. [Abstract] [Google Scholar]
- Pecoraro N, Reyes F, Gomez F, Bhargava A, Dallman MF. Chronic stress promotes palatable feeding, which reduces signs of stress: feedforward and feedback effects of chronic stress. Endocrinology. 2004;145:3754–3762. [Abstract] [Google Scholar]
- Pickens CL, Cifani C, Navarre BM, Eichenbaum H, Theberge FR, Baumann MH, Calu DJ, Shaham Y. Effect of fenfluramine on reinstatement of food seeking in female and male rats: implications for the predictive validity of the reinstatement model. Psychopharmacology (Berl) 2012;221:341–353. [Europe PMC free article] [Abstract] [Google Scholar]
- Ramachandrappa S, Farooqi IS. Genetic approaches to understanding human obesity. J Clin Invest. 2011;121:2080–2086. [Europe PMC free article] [Abstract] [Google Scholar]
- Ricart-Jane D, Rodriguez-Sureda V, Benavides A, Peinado-Onsurbe J, Lopez-Tejero MD, Llobera M. Immobilization stress alters intermediate metabolism and circulating lipoproteins in the rat. Metabolism. 2002;51:925–931. [Abstract] [Google Scholar]
- Richards JK, Simms JA, Steensland P, Taha SA, Borgland SL, Bonci A, Bartlett SE. Inhibition of orexin-1/hypocretin-1 receptors inhibits yohimbine-induced reinstatement of ethanol and sucrose seeking in Long-Evans rats. Psychopharmacology (Berl) 2008;199:109–117. [Europe PMC free article] [Abstract] [Google Scholar]
- Rodaros D, Caruana DA, Amir S, Stewart J. Corticotropin-releasing factor projections from limbic forebrain and paraventricular nucleus of the hypothalamus to the region of the ventral tegmental area. Neuroscience. 2007;150:8–13. [Abstract] [Google Scholar]
- Rowland NE, Antelman SM. Stress-induced hyperphagia and obesity in rats: a possible model for understanding human obesity. Science. 1976;191:310–312. [Abstract] [Google Scholar]
- Rutters F, Nieuwenhuizen AG, Lemmens SG, Born JM, Westerterp-Plantenga MS. Acute stress-related changes in eating in the absence of hunger. Obesity (Silver Spring) 2009;17:72–77. [Abstract] [Google Scholar]
- Rybkin, Zhou Y, Volaufova J, Smagin GN, Ryan DH, Harris RB. Effect of restraint stress on food intake and body weight is determined by time of day. Am J Physiol. 1997;273:R1612–1622. [Abstract] [Google Scholar]
- Salamone JD, Cousins MS, McCullough LD, Carriero DL, Berkowitz RJ. Nucleus accumbens dopamine release increases during instrumental lever pressing for food but not free food consumption. Pharmacol Biochem Behav. 1994;49:25–31. [Abstract] [Google Scholar]
- Sarlio-Lahteenkorva S, Rissanen A, Kaprio J. A descriptive study of weight loss maintenance: 6 and 15 year follow-up of initially overweight adults. Int J Obes Relat Metab Disord. 2000;24:116–125. [Abstract] [Google Scholar]
- Schachter S. Obesity and eating. Internal and external cues differentially affect the eating behavior of obese and normal subjects. Science. 1968;161:751–756. [Abstract] [Google Scholar]
- Schroeder BE, Schiltz CA, Kelley AE. Neural activation profile elicited by cues associated with the anxiogenic drug yohimbine differs from that observed for reward-paired cues. Neuropsychopharmacology. 2003;28:14–21. [Abstract] [Google Scholar]
- Scott C, Johnstone AM. Stress and Eating Behaviour: Implications for Obesity. Obes Facts. 2012;5:277–287. [Abstract] [Google Scholar]
- Shaham Y, Erb S, Stewart J. Stress-induced relapse to heroin and cocaine seeking in rats: a review. Brain Res Brain Res Rev. 2000;33:13–33. [Abstract] [Google Scholar]
- Shaham Y, Funk D, Erb S, Brown TJ, Walker CD, Stewart J. Corticotropin-releasing factor, but not corticosterone, is involved in stress-induced relapse to heroin-seeking in rats. J Neurosci. 1997;17:2605–2614. [Europe PMC free article] [Abstract] [Google Scholar]
- Shaham Y, Shalev U, Lu L, De Wit H, Stewart J. The reinstatement model of drug relapse: history, methodology and major findings. Psychopharmacology (Berl) 2003;168:3–20. [Abstract] [Google Scholar]
- Shepard JD, Bossert JM, Liu SY, Shaham Y. The anxiogenic drug yohimbine reinstates methamphetamine seeking in a rat model of drug relapse. Biol Psychiatry. 2004;55:1082–1089. [Abstract] [Google Scholar]
- Sinha R, Jastreboff AM. Stress as a common risk factor for obesity and addiction. Biol Psychiatry. 2013;73:827–835. [Europe PMC free article] [Abstract] [Google Scholar]
- Solomon MB, Foster MT, Bartness TJ, Huhman KL. Social defeat and footshock increase body mass and adiposity in male Syrian hamsters. Am J Physiol Regul Integr Comp Physiol. 2007;292:R283–290. [Abstract] [Google Scholar]
- Steffens AB, Strubbe JH. Regulation of body weight and food intake. Sci Prog. 1987;71:545–561. [Abstract] [Google Scholar]
- Sumithran P, Proietto J. The defence of body weight: a physiological basis for weight regain after weight loss. Clin Sci (Lond) 2013;124:231–241. [Abstract] [Google Scholar]
- Swiergiel AH, Wieczorek M. Noradrenaline-induced feeding responses in the rat do not depend on food characteristics. Acta Neurobiol Exp. 2008;68:354–361. [Abstract] [Google Scholar]
- Tellez LA, Ferreira JG, Medina S, Land BB, DiLeone RJ, de Araujo IE. Flavor-independent maintenance, extinction, and reinstatement of fat self-administration in mice. Biol Psychiatry. 2013;73:851–859. [Europe PMC free article] [Abstract] [Google Scholar]
- Torres SJ, Nowson CA. Relationship between stress, eating behavior, and obesity. Nutrition. 2007;23:887–894. [Abstract] [Google Scholar]
- Uhde TW, Boulenger JP, Post RM, Siever LJ, Vittone BJ, Jimerson DC, Roy-Byrne PP. Fear and anxiety: relationship to noradrenergic function. Psychopathology 17 Suppl. 1984;3:8–23. [Abstract] [Google Scholar]
- Valles A, Marti O, Garcia A, Armario A. Single exposure to stressors causes long-lasting, stress-dependent reduction of food intake in rats. Am J Physiol Regul Integr Comp Physiol. 2000;279:R1138–1144. [Abstract] [Google Scholar]
- Volkow ND, Wang GJ, Tomasi D, Baler RD. Obesity and addiction: neurobiological overlaps. Obes Rev. 2013;14:2–18. [Europe PMC free article] [Abstract] [Google Scholar]
- Wallach MB, Dawber M, McMahon M, Rogers C. A new anorexigen assay: stress-induced hyperphagia in rats. Pharmacol Biochem Behav. 1977;6:529–531. [Abstract] [Google Scholar]
- Wallis DJ, Hetherington MM. Emotions and eating. Self-reported and experimentally induced changes in food intake under stress. Appetite. 2009;52:355–362. [Abstract] [Google Scholar]
- Wanat MJ, Hopf FW, Stuber GD, Phillips PE, Bonci A. Corticotropin-releasing factor increases mouse ventral tegmental area dopamine neuron firing through a protein kinase C-dependent enhancement of Ih. J Physiol. 2008;586:2157–2170. [Abstract] [Google Scholar]
- Wellman PJ. Modulation of eating by central catecholamine systems. Current drug targets. 2005;6:191–199. [Abstract] [Google Scholar]
- Westerterp KR, Speakman JR. Physical activity energy expenditure has not declined since the 1980s and matches energy expenditures of wild mammals. Int J Obes (Lond) 2008;32:1256–1263. [Abstract] [Google Scholar]
- Williams KW, Elmquist JK. From neuroanatomy to behavior: central integration of peripheral signals regulating feeding behavior. Nat Neurosci. 2012;15:1350–1355. [Europe PMC free article] [Abstract] [Google Scholar]
- Wirtshafter D, Stratford TR. Evidence for motivational effects elicited by activation of GABA-A or dopamine receptors in the nucleus accumbens shell. Pharmacol Biochem Behav. 2010;96:342–346. [Europe PMC free article] [Abstract] [Google Scholar]
- Woods SC, Seeley RJ, Porte D, Jr, Schwartz MW. Signals that regulate food intake and energy homeostasis. Science. 1998;280:1378–1383. [Abstract] [Google Scholar]
- Zarrindast MR, Homayoun H, Babaie A, Etminani A, Gharib B. Involvement of adrenergic and cholinergic systems in nicotine-induced anxiogenesis in mice. Eur J Pharmacol. 2000;407:145–158. [Abstract] [Google Scholar]
- Zellner DA, Loaiza S, Gonzalez Z, Pita J, Morales J, Pecora D, Wolf A. Food selection changes under stress. Physiol Behav. 2006;87:789–793. [Abstract] [Google Scholar]
- Zheng H, Lenard NR, Shin AC, Berthoud HR. Appetite control and energy balance regulation in the modern world: reward-driven brain overrides repletion signals. Int J Obes (Lond) 2009;33(Suppl 2):S8–13. [Europe PMC free article] [Abstract] [Google Scholar]
- Zislis G, Desai TV, Prado M, Shah HP, Bruijnzeel AW. Effects of the CRF receptor antagonist D-Phe CRF(12-41) and the alpha2-adrenergic receptor agonist clonidine on stress-induced reinstatement of nicotine-seeking behavior in rats. Neuropharmacology. 2007;53:958–966. [Europe PMC free article] [Abstract] [Google Scholar]
Full text links
Read article at publisher's site: https://doi.org/10.1007/s00213-014-3838-1
Read article for free, from open access legal sources, via Unpaywall:
https://www.ncbi.nlm.nih.gov/pmc/articles/PMC4433618
Citations & impact
Impact metrics
Citations of article over time
Alternative metrics

Discover the attention surrounding your research
https://www.altmetric.com/details/3019173
Article citations
A neuronal coping mechanism linking stress-induced anxiety to motivation for reward.
Sci Adv, 9(49):eadh9620, 06 Dec 2023
Cited by: 2 articles | PMID: 38055830 | PMCID: PMC10699782
Opposite effects of stress on effortful motivation in high and low anxiety are mediated by CRHR1 in the VTA.
Sci Adv, 8(12):eabj9019, 23 Mar 2022
Cited by: 13 articles | PMID: 35319997 | PMCID: PMC8942367
Obesity and dietary fat influence dopamine neurotransmission: exploring the convergence of metabolic state, physiological stress, and inflammation on dopaminergic control of food intake.
Nutr Res Rev, 35(2):236-251, 28 Jun 2021
Cited by: 15 articles | PMID: 34184629 | PMCID: PMC9351269
Review Free full text in Europe PMC
Chronic Piromelatine Treatment Alleviates Anxiety, Depressive Responses and Abnormal Hypothalamic-Pituitary-Adrenal Axis Activity in Prenatally Stressed Male and Female Rats.
Cell Mol Neurobiol, 42(7):2257-2272, 18 May 2021
Cited by: 5 articles | PMID: 34003403
Brain mechanisms mediating effects of stress on reward sensitivity.
Curr Opin Behav Sci, 22:106-113, 22 Feb 2018
Cited by: 44 articles | PMID: 30349872 | PMCID: PMC6195323
Go to all (7) article citations
Similar Articles
To arrive at the top five similar articles we use a word-weighted algorithm to compare words from the Title and Abstract of each citation.
The anxiogenic drug yohimbine reinstates palatable food seeking in a rat relapse model: a role of CRF1 receptors.
Neuropsychopharmacology, 31(10):2188-2196, 07 Dec 2005
Cited by: 111 articles | PMID: 16341025 | PMCID: PMC1570156
Role of food type in yohimbine- and pellet-priming-induced reinstatement of food seeking.
Physiol Behav, 88(4-5):559-566, 27 Jun 2006
Cited by: 33 articles | PMID: 16806322 | PMCID: PMC1586114
The CRF1 receptor antagonist antalarmin attenuates yohimbine-induced increases in operant alcohol self-administration and reinstatement of alcohol seeking in rats.
Psychopharmacology (Berl), 195(3):345-355, 20 Aug 2007
Cited by: 138 articles | PMID: 17705061
Stress as a common risk factor for obesity and addiction.
Biol Psychiatry, 73(9):827-835, 26 Mar 2013
Cited by: 251 articles | PMID: 23541000 | PMCID: PMC3658316
Review Free full text in Europe PMC
Funding
Funders who supported this work.
NIDA NIH HHS (4)
Grant ID: R01DA037277
Grant ID: R01DA017288
Grant ID: R01 DA017288
Grant ID: R01 DA037277