Abstract
Free full text
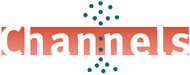
Correlations in timing of sodium channel expression, epilepsy, and sudden death in Dravet syndrome
Abstract
Dravet Syndrome (DS) is an intractable genetic epilepsy caused by loss‐of‐function mutations in SCN1A, the gene encoding brain sodium channel Nav1.1. DS is associated with increased frequency of sudden unexpected death in humans and in a mouse genetic model of this disease. Here we correlate the time course of declining expression of the murine embryonic sodium channel Nav1.3 and the rise in expression of the adult sodium channel Nav1.1 with susceptibility to epileptic seizures and increased incidence of sudden death in DS mice. Parallel studies with unaffected human brain tissue demonstrate similar decline in Nav1.3 and increase in Nav1.1 with age. In light of these results, we introduce the hypothesis that the natural loss Nav1.3 channel expression in brain development, coupled with the failure of increase in functional Nav1.1 channels in DS, defines a tipping point that leads to disinhibition of neural circuits, intractable seizures, co‐morbidities, and premature death in this disease.
Introduction
Dravet Syndrome (DS) is a progressive, infantile onset epileptic encephalopathy caused by heterozygous loss of function of SCN1A, the gene encoding the brain voltage sensitive sodium channel Nav1.1. DS begins with febrile seizures in infancy and progresses with development to include refractory generalized tonic‐clonic (GTC) seizures and other complex seizure types. Individuals with DS develop additional comorbid conditions including cognitive impairment, ataxia, psychomotor regression, and increased risk of premature death.1 Sudden unexpected death in epilepsy (SUDEP) is the leading cause of death in individuals with refractory epilepsy and constitutes a constant threat to patients with DS and their families.2,3 Risk of SUDEP is correlated with higher frequency of GTC seizures.4
Mice bearing a heterozygous loss‐of‐function DS mutation recapitulate the sensitivity to temperature‐induced seizures, progressive increase in seizure severity, cognitive impairment, comorbidities, and premature death of this disease.5-8 This DS mutation causes specific loss of Na+ currents and impaired excitability of GABAergic interneurons.8 SUDEP in these mice is caused by profound bradycardia due to increased parasympathetic output to the heart, immediately following a severe GTC seizure.9 Paralleling human SUDEP, all spontaneous deaths in DS mice occurred immediately following a GTC seizure, and the frequency of seizures and premature deaths rose sharply after spontaneous seizures began.9 Targeted deletion of Nav1.1 channels in forebrain specific GABAergic interneurons using the Cre‐Lox method recapitulates the susceptibility to seizures, co‐morbidities, and premature death of this disease,10 indicating that this specific deletion of Nav1.1 channels is sufficient to cause seizures, severe bradycardia, and premature death analogous to DS mice.9
Individuals with DS develop normally from birth until the time of initial seizure presentation at 5 to 11 mo.1 Similarly, DS mice are indistinguishable from their wild‐type littermates at birth and develop normally through the third week of life. During the fourth postnatal week, DS mice become susceptible to thermally induced and spontaneous seizures as well as premature death.7,8 Susceptibility to thermally induced seizures, spontaneous seizures, and SUDEP begins near the time of weaning in both mouse (P21) and human (6‐9 mo), suggesting that disease onset occurs at a similar stage of development in both species. This timing of disease onset and progression in human and mouse DS suggests a common underlying molecular event may trigger disease onset.
Nav1.1 is a member of a family of voltage‐sensitive sodium channels expressed primarily in the brain, including Nav1.1, Nav1.2, Nav1.3, and Nav1.6.11 In rat, Nav1.1, Nav1.2, and Nav1.6 channel expression increases continually from birth, while Nav1.3 channel expression is highest just prior to birth and declines in early postnatal life.12-15 Nav1.1 and Nav1.3 are both found primarily in the cell soma and proximal dendrites, but have complementary temporal expression patterns.16-18 In humans, Nav1.3 protein remains detectable in adult brain,19 but the developmental time course of expression of these channels remains unknown. In the experiments described here, we have determined the levels of Nav1.1 and Nav1.3 proteins in developing mouse and human brain and correlated these measurements with the onset of seizures and premature death in the 2 species.
Results and Discussion
Time course of expression of Nav1.1 and Nav1.3 channels in mouse brain
Previous studies of rat brain showed Nav1.3 mRNA and protein is expressed at high levels in embryonic life and declines in the first 3 weeks after birth, whereas Nav1.1 channel expression is first observed in early postnatal development and reaches its maximum expression level by 4 weeks of age.13,14 Immunoblotting of membrane proteins isolated from wild‐type C57Bl/6 mouse cerebral cortex showed an expression pattern similar to that seen in rat (Fig. 1A). In comparison with rat, the decline in Nav1.3 channel protein was more rapid, reaching its lowest levels at postnatal day 21 (P21), and the rise in Nav1.1 channels occurred later and the level of Nav1.1 was still increasing at P30 (Fig. 1A). Susceptibility to thermally induced seizures begins at P20 and there is an increase in the frequency of spontaneous seizures and death between P20 to P25 (Fig. 1B).7,9 By this time the level of Nav1.3 channel protein has declined to baseline, and the level of Nav1.1 channel protein is rapidly rising. In DS mice, the rise in functional Nav1.1 channel protein would be substantially reduced by the loss‐of-function mutations in 1 allele of the Scn1a gene.

Figure 1. Expression of sodium channel proteins in developing mouse cerebral cortex. (A) Sodium channel expression in membrane proteins from normal C57Bl/6 mouse cortex. Samples are expressed as a fraction of max expression after normalizing to β-actin. Data points represented as mean ± SEM, closed squares, Nav1.1; open triangles, Nav1.3. (B) Time course of spontaneous seizures (right axis) and premature death (left axis) in DS mice. Adapted from Kalume et al.9 with permission.
Time course of expression of Nav1.1 and Nav1.3 channels in human brain
Children diagnosed with DS develop normally until the time of seizure onset, which occurs between 5 and 11 mo, with an average age of onset of 5.7 mo (Table 1).1 After initial seizure onset, DS progresses over 1 to 2 years to multiple forms of spontaneous seizures, including generalized tonic-clonic seizures, resistance to drug therapy, developmental delay, and permanent mental and physical deficiencies.20-24 Following the onset of increased seizure incidence, the risk of SUDEP is also elevated with the majority of unexpected deaths occurring during the first 3 years of life.25
SCN1A mutation | Number of cases | Age of onset (months) | Citation |
+ | 32 | 5.6 ± 2.1 | Ragona, et al. 201020 |
- | 5 | 6.2 ± 2.4 | |
+ | 20 | 5.6 ± 2.1 | Ragona, et al.21 |
- | 6 | 5.8 ± 2.6 | |
+ | 29 | 5.7 ± 2.4 | Lim, et al. 201122 |
+ | 18 | 5.6 ± 2.3 | Petrelli, et al. 201123 |
- | 7 | 7.3 ± 4.2 | |
+ | 7 | 5.7 ± 1.9 | Chieffo, 201124 |
- | 5 | 5.0 ± 1.1 | |
Average onset: | 5.7 ± 2.3 |
+, indicates confirmed SCN1A mutation; -, denotes no SCN1A mutation detected. Compilation of age of initial seizure onset in patients diagnosed with Dravet Syndrome. Studies were chosen that identified mutations present in SCN1A in addition to behavioral characteristics. Mutation positive (+) and mutation negative (‐) were tabulated separately and reported.20-24
In order to compare sodium channel expression patterns during development of human brain, membrane proteins were isolated from non‐epileptic human postmortem cerebral cortex between 1.5 and 30 mo. Immunoblotting with subtype‐specific antibodies showed Nav1.1 protein expression parallels rodent expression, with low levels of Nav1.1 at birth, steadily increasing to its highest level by 20 mo (Fig. 2). Human Nav1.3 protein expression also parallels the rodent pattern and is detected at its highest levels at birth, decreasing to its lowest level at 6 mo of age (Fig. 2). The time at which the decline of Nav1.3 protein levels and the rise of Nav1.1 expression cross (approximately 5‐6 mo) correlates closely with the mean time of seizure onset in patients with DS (approximately 6 mo; Table 1). The complementary time courses of expression Nav1.1 and Nav1.3 coupled with their similar localization in neuronal cell bodies suggest that these channels may perform similar functions and that Nav1.3 may be protective against loss of Nav1.1 channels at early ages.

Figure 2. Expression of sodium channel proteins in developing human cerebral cortex. Channel expression in membrane proteins from normal postmortem human cortical tissue. Samples are represented as a fraction of max expression after normalizing to β‐tubulin; closed squares, Nav1.1; open triangles, Nav1.3.
Complementary Expression of Nav1.1 and Nav1.3 Define a Tipping Point in DS
The results of our experiments demonstrate that the decline of Nav1.3 and the rise of Nav1.1 expression in human cerebral cortex occur in the same time frame as the initial presentation of seizures in patients diagnosed with DS, both at around 6 mo (Fig. 2). Similarly, the timing of the decline of Nav1.3 and the rise of Nav1.1 expression in rodents occur in the third and fourth week of life (Fig. 1A),13 coincident with susceptibility of our DS mouse model to thermally induced seizures, spontaneous seizures, and SUDEP.7-9 These data in human, rat, and mouse suggest that the timing of DS onset is a result of the failure of Nav1.1 channels to fully replace the normal developmental loss of embryonic Nav1.3 channels.
In previous work,8 we used immuncytochemical methods to assess possible up‐regulation of Nav1.3 channels in Nav1.1+/‐ and Nav1.1‐/‐ mice at P14, when the level of Nav1.3 has decreased to approximately 10% of maximum in cerebral cortex of WT mice (Fig. 1). We found no up‐regulation of Nav1.3 channels in hippocampal interneurons of Nav1.1+/‐ mice, our DS model. There was significant up‐regulation of Nav1.3 channels in hippocampal interneurons of Nav1.1‐/‐ mice, but the level was insufficient to rescue either the sodium currents or the epileptic phenotype of these mice.8 We found no up‐regulation of Nav1.2, Nav1.3, or Nav1.6 channels in excitatory or inhibitory neurons in immunocytochemical analyses of cerebral cortex, thalamus, or cerebellum of Nav1.1‐/‐ mice. Thus, surprisingly, there is little compensatory up‐regulation of Nav1.3 or other sodium channels in mouse forebrain, even in the face of complete deletion of Nav1.1 channels.
In light of the complementary time courses of expression of Nav1.1 and Nav1.3 channels, the loss of Nav1.3 channel expression may be one of the precipitating factors that initiates disease onset in DS, and Nav1.3 may also function as a genetic modifier that contributes to the striking genetic background effects in DS in mice and humans. The time of crossover of declining levels of Nav1.3 channels and rising levels of Nav1.1 channels (Figs. 1 and and2)2) may define the tipping point for development of DS, as the mutations characteristic of this disease prevent the normal increase in functional Nav1.1 channels in forebrain GABAergic inhibitory neurons leading to disinhibition of neural circuits, epilepsy, neurodevelopmental co‐morbidities, and SUDEP.
Methods
Immunoblotting
Normal postmortem human brain samples were obtained from the NICHD Brain and Tissue Band for Developmental Disorders at the University of Maryland. Ages analyzed were 1.4, 1.8, 5.2, 5.6, 6, 6.8, 10.7, 20.8, 30.3 mo. Immunoblotting of human tissue was done at Seattle Children’s Research Institute as previously described in detail.26 Briefly, membrane proteins were isolated from 100‐200 μg of cortical tissue cleaved from the underlying white matter, transferred to PVDF membranes and probed for Nav1.1 (1/200, rabbit anti‐Nav1.1, Alomone labs), Nav1.3 (1/200, rabbit anti‐Nav1.3, Alomone labs) and β‐tubulin (1/10,000, mouse anti‐tubulin, Novus Biologicals). Infrared fluorescence was used for signal detection and quantitation (rabbit anti‐Alexa Fluor 680, Molecular Probes; IRDye 800 goat antimouse IgG, LI‐COR; Odyssey Infrared Imagins System, LI‐COR Biosciences). Background was subtracted, and levels of Nav1.1 α subunit protein are represented as relative expression to β‐tubulin.
Mouse cortical tissues were dissected from WT C57Bl/6 animals (Jackson Laboratories) at postnatal days (P) 1, 4, 7, 10, 14, 17, 21, and 30 to represent a wide range of developmental ages. Following membrane protein isolation as previously described.26 Pelleted membrane proteins were resuspended in 1x RIA buffer (25 mM Tris, 150 mM NaCl, 1 mM EDTA, 2% Triton X‐100, pH 7.4) and centrifuged at 20,000xg for 20 min, and the supernatant containing the membrane proteins was collected for analysis. Complete Protease Inhibitor set (Roche) was included in all solutions. Protein concentration was determined by BCA assay (Pierce) and transferred to nitrocellulose membranes and immunoblotted as previously described27 with antibodies specific for Nav1.1 (1/200, anti‐rabbit Nav1.1, Alomone labs), Nav1.3 (1/100, Sp32iii)18 and β‐actin (1/10,000, anti‐mouse β actine, Abcam Inc.). Samples were background subtracted and are represented as relative expression to β‐actin normalized to the maximum expression for each channel ± SEM, n = 3 for each time point represented.
Animal care and analysis
All animal procedures were approved by the Institutional Animal Care and Use Committee of the University of Washington.
Acknowledgments
This work was supported by National Institutes of Health Research Grants R01 NS25704 (Catterall WA), K02 NS072162 (Jansen LA), K01 NS062862 (Kalume FK) and K08 NS071193 (Oakley JC).
Disclosure of Potential Conflicts of Interest
No potential conflicts of interest were disclosed.
Footnotes
Previously published online: www.landesbioscience.com/journals/channels/article/26023
References
Articles from Channels are provided here courtesy of Taylor & Francis
Full text links
Read article at publisher's site: https://doi.org/10.4161/chan.26023
Read article for free, from open access legal sources, via Unpaywall:
https://www.tandfonline.com/doi/pdf/10.4161/chan.26023?needAccess=true
Citations & impact
Impact metrics
Citations of article over time
Alternative metrics

Discover the attention surrounding your research
https://www.altmetric.com/details/3822341
Article citations
Mechanisms of neurodevelopmental toxicity of topiramate.
Crit Rev Toxicol, 54(7):465-475, 12 Jul 2024
Cited by: 0 articles | PMID: 38995641
Review
AARS and CACNA1A mutations: diagnostic insights into a case report of uncommon epileptic encephalopathy phenotypes in two siblings.
Front Neurol, 15:1376643, 15 Apr 2024
Cited by: 0 articles | PMID: 38689878 | PMCID: PMC11059961
Targeted blockade of aberrant sodium current in a stem cell-derived neuron model of SCN3A encephalopathy.
Brain, 147(4):1247-1263, 01 Apr 2024
Cited by: 1 article | PMID: 37935051 | PMCID: PMC10994535
Channelopathies in epilepsy: an overview of clinical presentations, pathogenic mechanisms, and therapeutic insights.
J Neurol, 271(6):3063-3094, 12 Apr 2024
Cited by: 2 articles | PMID: 38607431
Review
A structurally precise mechanism links an epilepsy-associated <i>KCNC2</i> potassium channel mutation to interneuron dysfunction.
Proc Natl Acad Sci U S A, 121(3):e2307776121, 09 Jan 2024
Cited by: 6 articles | PMID: 38194456 | PMCID: PMC10801864
Go to all (37) article citations
Data
Similar Articles
To arrive at the top five similar articles we use a word-weighted algorithm to compare words from the Title and Abstract of each citation.
A selective NaV1.1 activator with potential for treatment of Dravet syndrome epilepsy.
Biochem Pharmacol, 181:113991, 23 Apr 2020
Cited by: 12 articles | PMID: 32335140
Cardiac-Specific Deletion of Scn8a Mitigates Dravet Syndrome-Associated Sudden Death in Adults.
JACC Clin Electrophysiol, 10(5):829-842, 28 Feb 2024
Cited by: 1 article | PMID: 38430092 | PMCID: PMC11285447
Atypical myelinogenesis and reduced axon caliber in the Scn1a variant model of Dravet syndrome: An electron microscopy pilot study of the developing and mature mouse corpus callosum.
Brain Res, 1751:147157, 15 Oct 2020
Cited by: 5 articles | PMID: 33069731
Sudden unexpected death in Dravet syndrome: respiratory and other physiological dysfunctions.
Respir Physiol Neurobiol, 189(2):324-328, 09 Jul 2013
Cited by: 34 articles | PMID: 23850567 | PMCID: PMC7213775
Review Free full text in Europe PMC
Funding
Funders who supported this work.
NINDS NIH HHS (5)
Grant ID: R01 NS25704
Grant ID: K02 NS072162
Grant ID: R01 NS025704
Grant ID: K01 NS062862
Grant ID: K08 NS071193