Abstract
Background
Diffuse intrinsic pontine glioma (DIPG) is a fatal disease with a median overall survival (OS) of less than 12 months after diagnosis. Radiotherapy (RT) still remains the mainstay treatment. Several other therapeutic strategies have been attempted in the last years without a significant effect on OS. Although radiological imaging is the gold standard for DIPG diagnosis, the urgent need to improve the survival has led to the reconsideration of biopsy with the aim to better understand the molecular profile of DIPG and support personalized treatment.Methods
In this study, we present a single-center experience in treating DIPG patients at disease progression combining targeted therapies with standard of care. Biopsy was proposed to all patients at diagnosis or disease progression. First-line treatment included RT and nimotuzumab/vinorelbine or temozolomide. Immunohistochemistry-targeted research included study of mTOR/p-mTOR pathway and BRAFv600E. Molecular analyses included polymerase chain reaction, followed by Sanger sequences and/or next-generation sequencing.Results
Based on the molecular profile, targeted therapy was administered in 9 out of 25 patients, while the remaining 16 patients were treated with standard of care. Personalized treatment included inhibition of the PI3K/AKT/mTOR pathway (5/9), PI3K/AKT/mTOR pathway and BRAFv600E (1/9), ACVR1 (2/9) and PDGFRA (1/9); no severe side effects were reported during treatment. Response to treatment was evaluated according to Response Assessment in Pediatric Neuro-Oncology criteria, and the overall response rate within the cohort was 66%. Patients treated with targeted therapies were compared with the control cohort of 16 patients. Clinical and pathological characteristics of the two cohorts were homogeneous. Median OS in the personalized treatment and control cohort was 20.26 and 14.18 months, respectively (p = 0.032). In our experience, the treatment associated with the best OS was everolimus.Conclusion
Despite the small simple size of our study, our data suggest a prognostic advantage and a safe profile of targeted therapies in DIPG patients, and we strongly advocate to reconsider the role of biopsy for these patients.Free full text

Targeted therapy for pediatric diffuse intrinsic pontine glioma: a single-center experience
Abstract
Background:
Diffuse intrinsic pontine glioma (DIPG) is a fatal disease with a median
overall survival (OS) of less than 12months after diagnosis. Radiotherapy
(RT) still remains the mainstay treatment. Several other therapeutic
strategies have been attempted in the last years without a significant
effect on OS. Although radiological imaging is the gold standard for DIPG
diagnosis, the urgent need to improve the survival has led to the
reconsideration of biopsy with the aim to better understand the molecular
profile of DIPG and support personalized treatment.
Methods:
In this study, we present a single-center experience in treating DIPG patients at disease progression combining targeted therapies with standard of care. Biopsy was proposed to all patients at diagnosis or disease progression. First-line treatment included RT and nimotuzumab/vinorelbine or temozolomide. Immunohistochemistry-targeted research included study of mTOR/p-mTOR pathway and BRAFv600E. Molecular analyses included polymerase chain reaction, followed by Sanger sequences and/or next-generation sequencing.
Results:
Based on the molecular profile, targeted therapy was administered in 9 out of
25 patients, while the remaining 16 patients were treated with standard of
care. Personalized treatment included inhibition of the PI3K/AKT/mTOR
pathway (5/9), PI3K/AKT/mTOR pathway and BRAFv600E (1/9),
ACVR1 (2/9) and PDGFRA (1/9); no
severe side effects were reported during treatment. Response to treatment
was evaluated according to Response Assessment in Pediatric Neuro-Oncology
criteria, and the overall response rate within the cohort was 66%. Patients
treated with targeted therapies were compared with the control cohort of 16
patients. Clinical and pathological characteristics of the two cohorts were
homogeneous. Median OS in the personalized treatment and control cohort was
20.26 and 14.18months, respectively (p
=
0.032). In our
experience, the treatment associated with the best OS was everolimus.
Conclusion:
Despite the small simple size of our study, our data suggest a prognostic advantage and a safe profile of targeted therapies in DIPG patients, and we strongly advocate to reconsider the role of biopsy for these patients.
Introduction
Diffuse intrinsic pontine glioma (DIPG), accounting for about 10% of all brain
malignancies, is the deadliest pediatric central nervous system tumor.1,2 The median progression-free
survival (PFS) is 7months and less than 10% of the patients survive 2
years after
diagnosis.3,4
Magnetic resonance imaging (MRI) is the gold standard for DIPG diagnosis. 5 Typical MRI findings reveal a large expansive pontine lesion, occupying more than 50% of its ventral portion that appears hypointense or isointense on T1-weighted (T1w) imaging, and hyperintense on T2-weighted (T2w) imaging and on fluid-attenuated inversion recovery (FLAIR) imaging. 5
Radiotherapy (RT) remains the mainstay treatment for DIPG, a clinical and
radiological response being observed in about 70% of patients
6
; meanwhile, several chemotherapeutic strategies have been explored in
different clinical trials during the last 30years, but, unfortunately, none of them
were shown to induce complete remission of the disease.3,7–12
To date, standardized therapy has improved PFS and time to progression (TTP) with no
impact on overall survival (OS) in these patients.6,13 Recent study confirms a
median OS of 11months (interquartile range: 7.5–16).
14
Analysis on autoptic samples demonstrated that DIPGs present an
overexpression/activation of epidermal growth factor receptor (EGFR) protein.
Investigating EGFR protein role, Massimino et al.
15
proposed the clinical use of anti-EGFR monoclonal antibody, nimotuzumab, in
combination with RT and vinorelbine as a potential synergic treatment strategy,
reporting the best OS with a median time of 15months in patients treated with this
approach.
Tumor biopsy is not mandatory for DIPG diagnosis and is currently indicated in the presence of atypical radiological features or in the setting of a clinical trial. 16 The role of biopsy is increasingly being redefined over time, enabling to conduct biology-driven translational research, supporting target therapy, and allowing advanced clinical trial studies, as well as contributing to better detail the disease diagnosis. 17
More than 80% of DIPGs harbor a mutation in the H3 genes, especially in the N-terminal tail of histone H3, with a substitution of lysine at position 27. 18 The H3K27M mutation and the loss of H3K27me3 are the paramount features of DIPG. Molecular studies have unveiled numerous shared genetic alterations, with a striking prevalence of the K27M mutation in genes encoding the histones H3.3 and H3.1, mutually exclusive in DIPG. 18
Additional recurrent mutations have also been identified. Mutations in ACVR1 co-occur in 20–30% of H3-mutated DIPG, and mutations in TP53 are found in up to 77% of these tumors. 19
Since the discovery of the K27M mutation in the H3 histone genes,20,21 many co-segregating mutations have been unveiled, 22 on which much effort has been made in exploring the possibility of targeted therapeutic approaches. 23
Here, we present our experience in treating DIPG patients at the time of disease progression, combining targeted therapy to our institutional second-line treatment and discuss the benefit of such approach.
Materials and methods
This study includes children with a diagnosis of DIPG who were admitted to Bambino Gesù Children’s Hospital in Rome from 2014 to 2019.
Radiology
MRI examinations were performed on a 3 Tesla (3 T) magnet (Siemens Magnetom
Vision Plus, c Erlangen, Germany), and general anesthesia was utilized for
non-cooperating children. The MRI was extended to whole brain and spinal cord,
with at least T1w sagittal, T1w and T2w axial sequences, FLAIR, T2w coronal and
diffusion-weighed imaging (DWI) sequences. Acquisition protocols employed 3mm
of slice thickness.
5
Radiological response during treatment was evaluated by an experienced
neuroradiologist (GSC) in accordance with the response assessment in
neuro-oncology (RANO) criteria.
24
To confirm treatment response according to the most recent Response
Assessment in Pediatric Neuro-Oncology (RAPNO) criteria
25
specific for DIPG, all cases were retrospectively reviewed by a blinded
experienced neuroradiologist (GSC).
Frameless stereotactic biopsy
Biopsy was proposed to all patients at the time of diagnosis or at first progression. Robot-assisted stereotactic needle biopsy was performed using a trans-frontal approach as previously described by our group. 26 Biopsy samples were used for diagnostic and translational research purposes, according to the following prioritization: (a) histology and immunostaining for H3K27M; (b) molecular analysis: Sanger sequencing or next-generation sequencing (NGS); (c) immunohistochemistry (IHC) target detection; and (d) establishment of cell cultures.
Pathology and IHC
IHC was carried out on formalin-fixed paraffin-embedded sections using an automated immunostainer (Dako Omnis, Agilent, Santa Clara, CA USA). Primary antibodies directed against the following antigens were applied: ATRX (clone AX1, 1/100, high pH, Dianova, Hamburg, Germany), GFAP (polyclonal, prediluted, high pH, Dako, Santa Clara, USA), H3K27M (polyclonal, 1/1500, high pH, Millipore, Danvers, Massachusetts, USA), H3.3K27me3 (clone C36B11, 1/200, low pH, Cell Signaling technology), Ki67 (clone MIB-1, prediluted, low pH, Dako, Santa Clara, USA), OLIG2 (1/20, high pH, Quartett, Schichauweg, Berlin, Germany), p53 (clone DO-7, prediluted, high pH, Dako, Santa Clara, USA), BRAFv600E (clone VE1, 1/50, high pH, Spring Bioscience Corp.,4300 Hacienda Dr, Pleasanton, CA 94588, USA), mTOR (clone 7C10 1:50, high pH, Cell Signaling Technology, Danvers, Massachusetts, USA) and p-mTORSer2448 (D9C2, 1:100, high pH, Cell Signaling Technology, Danvers, Massachusetts, USA). P53 was interpreted as overexpressed when nuclear staining was seen in 50% of the neoplastic cells or more. Ki67 was expressed as percentage of positive neoplastic cells.
DNA extraction from snap frozen tissue and Sanger sequencing
DNA was extracted from snap frozen tissue following the DNeasy Blood & Tissue kit protocol (Qiagen, Germany), while for FFPE tumor tissue, cores were taken from tumor area and used for genomic DNA isolation, following NucleoSpin tissue kit protocol (Macherey-Nagel). DNA concentration was determined on a Nanodrop 2000 (Thermo Fisher, Waltham, Massachusetts, USA). Polymerase chain reaction (PCR) was carried out as previously described 27 to analyze hotspot mutations for the following genes: H3F3A (NM_002107), HIST1H3B (NM_003537), HIST1H3C (NM_003531), and HIST2H3C (NM_003531), along with exons 4–7 of ACVR1 (NM_001105), using primers obtained from Sigma Aldrich (St. Louis, Missouri, USA). 27
Products were purified using NucleoSpin Gel and PCR Clean-up (Macherey-Nagel, Germany), quantified on a Nanodrop 2000 (Thermo Scientific, Waltham, Massachusetts, USA) and subjected to bidirectional sequencing using BigDye Terminator v3.1 (Applied Biosystem™, Waltham, Massachusetts, USA). After purification with Nucleoseq (Macherey Nagel, Germany), capillary sequencing was performed on a 3500 Genetic analyser (Applied Biosytem™, Waltham, Massachusetts, USA). Sequences were analyzed using the Mutation Surveyor (SoftGenetics, Pennsylvania, USA) and manually with FinchTV (Geospiza, Seattle, USA). Mutations were called when an aberrant peak was clearly distinguishable from background noise in both the forward and reverse sequence, or if an aberrant peak was identified by the software in at least one of the sequences and confirmed by visual inspection. The new putative variants identified were compared with the somatic mutations listed in the COSMIC (https://cancer.sanger.ac.uk/cosmic), VarSome (https://varsome.com/) and dbSNP (https://www.ncbi.nlm.nih.gov/snp/) databases. Silico tools such as PolyPhen-2 (http://genetics.bwh.harvard.edu/pph2/) and Provean (http://provean.jcvi.org/index.php) were used to predict whether an amino acid substitution had an impact on the biological function of the protein ACVR1.
Next-generation sequencing
DNA sequencing was performed by means of an IDT-xGen Predesigned Gene Capture Panel (Integrated DNA Technologies, Leuven, Belgium) for a total of 336 genes, of which 99 genes reportedly mutated in pediatric high-grade gliomas and other pediatric brain tumors;28–30 and the others were used to investigate cancer predisposition syndromes. DNA libraries were prepared using the Nextera™ DNA Flex Library Prep (Ilumina, San Diego, CA, USA) according to the manufacturer’s protocols, and sequencing was done on a NextSeq 550 (Illumina). The fastq files were aligned to the human reference GRCh37/hg19. The BWA Enrichment application of BaseSpace (Illumina) and the TGex software (LifeMap Sciences) were used for the calling and annotating variants, respectively. Sequence data were carefully analyzed, and the presence of all suspected variants was checked in the public databases [dbSNP; http://www.ncbi.nlm.nih.gov/projects/SNP), 1000 Genomes Project (http://www.internationalgenome.org/), EVS (http://evs.gs.washington.edu/EVS/), ExAC (http://exac.broadinstitute.org/), gnomAD (http://gnomad.broadinstitute.org/), and Cosmic (https://cancer.sanger.ac.uk/cosmic). Sequencing data filtering was performed to identify germline and somatic pathogenic or likely pathogenic variants with an allele frequency <1%, according to dbSNP’s overall frequency that results in a change in the amino acid sequence (i.e. missense/nonsense) or that reside within a putative splice site junction (with a window size of 7 bases). Functional impact of variants was analyzed by Combined Annotation Dependent Depletion V.1.3, Sorting Intolerant from Tolerant, and Polymorphism Phenotyping v2 (PolyPhen-2). Selected variants were interpreted in accordance with the American College of Medical Genetics and Genomics/Association for Molecular Pathology. Variants were examined for coverage and Q-score (minimum threshold of 30) and were visualized by the Integrative Genome Viewer.
Patient’s treatment
First-line treatment consisted of focal RT associated with nimotuzumab and vinorelbine.
15
Two patients had received temozolomide associated with RT at other
institutions, prior to admission to our hospital. Patient-adapted treatment
regimens were used at the time of progression. Re-irradiation was performed when
progression occurred at least 6months after previous RT.
31
Second-line chemotherapy included bevacizumab and temozolomide, or
nimotuzumab/vinorelbine, when not performed as first-line treatment.
At progression, based on their tumor molecular makeup, patients were assigned to a targeted treatment plan, in accordance with the multidisciplinary molecular tumor board’s consensus report. Targeted treatments included everolimus, vemurafenib, pazopanib, and palovarotene, which when appropriate were used individually or in combination. Posology was chosen according to the literature data and previously reported experience in the pediatric population.32–34 Drugs against selected targets were associated with concomitant chemotherapy in all cases. Treatment side effects were reported according to Common Terminology Criteria for Adverse Effects (AE) 5.0 version.
Statistical analysis
Patients were stratified in two groups according to treatment received: targeted versus non-targeted treatment. Comparison tests included Fisher’s exact test and Student’s t test distribution. OS was defined as the time from diagnosis to death, and survival after the first progression (SFP) was defined as the time from first progression of the disease to death. TTP was described in the target population as the time from start of targeted therapy to progression.
OS and SFP were calculated using the Kaplan–Meier method. Survival curves were compared with log-rank tests; multivariate analyses were not performed due to the limited sample sizes. All comparison tests were two-sided and considered significant at the 5% level. SAS 9.4 version software was used for statistical analysis.
Results
Between 2015 and 2019, we enrolled 25 DIPG patients at diagnosis or progression who were being treated at the Bambino Gesù Children’s Hospital. All patients fulfilled radiologic criteria for DIPG diagnosis.
Median age at diagnosis was 6.1years (range: 3.6–14.7), and 16/25 (64%) patients
were female. Main clinical and radiological characteristics according to the
treatment stratification (targeted versus other treatments) are
detailed in Table
1.
Table 1.
Patient, tumor, and first-line treatment characteristics.
Characteristics | Target therapy (n![]() ![]() | No-target therapy (n![]() ![]() |
---|---|---|
Gender | ||
![]() | 3:6 | 6:10 |
![]() | ||
![]() | 6.01![]() | 6.07![]() |
Duration of symptoms | ||
![]() | 3 (33%) | 5 (31%) |
![]() ![]() | 5 (56%) | 8 (50%) |
![]() ![]() | 1 (11%) | 3 (19%) |
Neurological signs | ||
![]() | 5 (56%) | 12 (75%) |
![]() | 3 (33%) | 3 (19%) |
![]() | 1 (11%) | 1 (6%) |
Radiological features | ||
![]() ![]() ![]() | 9 (100%) | 16 (100%) |
Tumor extension beyond the pons | ||
![]() | 1 (11%) | 0 |
![]() | 8 (89%) | 16 (100%) |
![]() | 5 (62.5%) | 6 (37.5%) |
![]() | 8 (100%) | 15 (94%) |
![]() | 0 | 4 (25%) |
![]() | 0 | 2 (12.5%) |
![]() | 0 | 4 (25%) |
Tumor morphology | ||
![]() | 6 (67%) | 14 (87.5%) |
![]() | 6 (67%) | 7 (44%) |
![]() | 9 (100%) | 15 (94%) |
Tumor signal | ||
![]() | 9 (100%) | 16 (100%) |
Enhancement | ||
![]() | 0 | 0 |
![]() | 1 (11%) | 3 (19%) |
![]() | 4 (45%) | 6 (37.5%) |
![]() | 2 (22%) | 3 (19%) |
![]() | 9 (100%) | 16 (100%) |
![]() | 9 (100%) | 11 (69%) |
![]() | 6 (67%) | 3 (19%) |
![]() | 4 (44%) | 2 (12.5%) |
Histological examination | ||
![]() | 9 (100%) | 16 (100%) |
First-line treatment | ||
![]() ![]() ![]() | 8 (89%) | 15 (94%) |
![]() ![]() ![]() | 1 (11%) | 1 (6%) |
TTP from end of RT | ||
![]() ![]() | 2 (22%) | 5 (31.5%) |
![]() ![]() | 6 (67%) | 10 (62.5%) |
>360![]() | 1 (11%) | 1 (6%) |
Re-irradiation at relapse | ||
![]() | 7 (1) | 10 (1) |
Second-line treatments | ||
![]() ![]() ![]() | 7 (78%) | 12 (75%) |
![]() ![]() ![]() | 1 (11%) | 1 (6%) |
![]() | 1 (11%) | 3 (19%) |
BVZ, bevacizumab; N/V, nimotuzumab/vinorelbine; RT, radiotherapy; TMZ, temozolomide; TTP, time to progression.
Metastatic disease at diagnosis was excluded on the basis of cerebrospinal fluid cytology and MRI in all cases.
Biopsy
Biopsy was performed in all cases. None of the patients showed hemorrhage at the
postoperative imaging and no irreversible neurological deficit was observed;
confirming biopsy safety. Only three patients presented transient neurologic
impairment after biopsy, completely resolved after maximum 2months. A flow
chart showing the investigation carried out on tumor samples is described in
Figure 1.
Collected samples allowed pathological diagnosis of DIPG in all cases:
(a)
Expression of glial markers, that is, GFAP and/or OLIG2, and the presence of the H3K27M mutation by the mutation-specific antibody was explored in all cases. IHC showed H3K27M mutation in 24/25 cases. H3.3K27me3 loss of expression was observed in 19 out of 20 patients tested; p53 was overexpressed in half of the cases analyzed (10/21); ATRX proved lost in about one-fourth of the cases tested (5/19). Ki67 expression varied from 5% to 60% (median 15%). As for the morphological features, all lesions showed an infiltrative growth pattern and the majority of tissue samples were hypercellular throughout (15/25); nuclear atypia scored as severe only in a minority of cases (3/25); mitoses were brisk in 7/20 cases. Histological and immunostaining features are detailed in Table 2.
Table 2.
Molecular characteristics of the DIPG patients’ cohort treated with or without target therapies.
Target therapy No target therapy Number (%) 9 (36%) 16 (64%) Histological and IHC findings Hypercellularity Yes, n (%)
5 (55.5) 10 (62.5) No, n (%)
4 (44.5) 6 (37.5) Data missing, n (%)
0 (0) (0) Mitoses Low, n (%)
4 (44.5) 9 (56) High, n (%)
3 (33.5) 4 (25) Data missing, n (%)
2 (22) (19) Atypia Mild, n (%)
4 (44.5) 8 (50) Moderate, n (%)
4 (44.5) 6 (37.5) Severe, n (%)
1 (11) 2 (12.5) Data missing, n (%)
0 (0) (0) Ki67 expression 5%, n (%)
3 (33.3) 1 (6.25) 5–15%, n (%)
3 (33.3) 8 (50) >15%, n (%)
2 (22.3) 5 (31.25) Data missing, n (%)
1 (11.1) (12.5) P53 expression >50%, n (%)
2 (22) 8 (50) <50%, n (%)
5 (56) 5 (31.25) Data missing, n (%)
2 (22) (18.75) ATRX Maintained, n (%)
5 (55.6) 9 (56.25) Lost, n (%)
2 (22.2) 3 (18.75) Data missing, n (%)
2 (22.2) (25) H3K27 me3 Maintained, n (%)
1 (11.1) 0 (0) Lost, n (%)
8 (88.9) 11 (68.75) Data missing, n (%)
0 (0) 5 (31.25) H3K27M IHC Positivity, n (%)
8 (89) 16 (100) Negativity, n (%)
1 (11) 0 (0) Data missing, n (%)
0 (0) (0) BRAF IHC Expressed, n (%)
1 (11) 0 (0) Not expressed, n (%)
4 (44,5) 10 (62.5) Data missing, n (%)
4 (44,5) (37.5) mTOR/pm-TOR IHC Expressed, n (%)
7 (78) 3 (19) Not expressed, n (%)
0 (0) 4 (25) Data missing, n (%)
2 (22) 9 (56) Molecular findings Histone mutation detection
8 (88.9) 16 (100) Type of mutation H3F3A, n (%)
3 (37.5) 11 (68.5) HIST1H3B, n (%)
5 (62.5) 4 (25) HIST2H3C, n (%)
0 (0) 1 (6.5) ACVR1 detection
8 (88.9) 16 (100) ACVR1 mutated, n (%) 4 (50) 4 (25) Wild-type, n (%)
4 (50) 12 (75) DIPG, diffuse intrinsic pontine glioma; IHC, immunohistochemistry.
(b)
The histone genes mutational status was investigated by Sanger sequencing or NGS in 24/25 (not performed in one case due to insufficient material). The K27M mutation was found in H3F3A for 14/24 cases (58.3%), in HIST1H3B for 9/24 patients (37.5%) and in HIST2H3C for 1/24 patients (4.2%). The only one case negative for H3K27M expression by IHC was found to be mutated by NGS. ACVR1 genes mutational status was investigated by Sanger sequencing or NGS in all but one case. ACVR1 was mutated in 8/24 cases (33.3%). NGS was performed globally in 14 cases (in 5 cases at the time of diagnosis and in 9 cases was retrospectively performed). Additional mutations found in NGS are reported in Figure 2. All detailed NGS mutation findings are listed in Supplemental material.
(c)
In the majority of the cases, the amount of tissue allowed for the evaluation of additional immunostainings, including those for therapeutic targets, that is, mTOR/p-mTOR (14/25) and BRAF-mutant (15/25). mTOR/p-mTOR pathway was overexpressed in 10/14 investigated (71%). BRAFv600E was positive in only 1/15 cases tested (7%), as confirmed by sequencing (Figure 2).
(d)
A tissue fragment was used whenever possible to set up cell cultures, allowing for the establishment of primary patient-derived cell lines in 17/25 cases (68%).
Treatment
In all, 23 patients presented to our center at the time of diagnosis and received
first-line treatment with RT (59Gy), nimotuzumab (150
mg/m2), and
vinorelbine (20
mg/m2) weekly for 12
weeks, followed by nimotuzumab
(150
mg/m2) and vinorelbine (25
mg/m2) every 2
weeks
until progression, with concomitant valproic acid (20
mg/kg/day). Two patients
who presented at the time of disease progression had been previously treated
with temozolomide (75
mg/m2 daily) in association to radiation
(54
Gy) as first-line treatment.
Re-irradiation, with a dose range of 10.8–36Gy, was performed in patients who
showed progression of disease more than 6
months after the last irradiation
(17/25) and was homogeneous for both groups.
Chemotherapeutic agents used at progression were humanized anti-vascular
endothelial growth factor monoclonal antibody bevacizumab (10mg/kg every
2
weeks) and temozolomide (with a dosage range of 150–250
mg/m2
5
days monthly). Nimotuzumab and vinorelbine were used in association with
bevacizumab in the two patients who received temozolomide as first-line
treatment.
In 15/25 cases (60%) for which tumor tissue was available for IHC and DNA
sequencing analysis, a number of potential targetable alterations were found.
Based on these findings, 9/15 patients were treated with targeted therapy (64%).
Six of 15 patients were not eligible for targeted therapy due to advanced stage
of disease. Among the patients who received a targeted treatment, identified
alterations included the following: mTOR/p-mTOR (5/9), ACVR1
(2/9), mTOR/p-mTOR and BRAFv600E (1/9), and
PDGFRA (1/9). Patients whose tumor tissue expressed
mTOR/p-mTOR by IHC were treated with everolimus (2.5mg/day under 30
kg of
bodyweight, 5
mg/day above 30
kg). Two patients whose tumor harbored an
ACVR1 mutation received palovarotene (20
mg/day). One child
was given vemurafenib (480
mg/day) in combination with everolimus due to the
coexistence of BRAFv600E mutation and mTOR/p-mTOR expression in
the tumor tissue. Pazopanib (200
mg/day) was used in a single case to target
PDGFRA. No severe AEs were described in all the patients
treated with targeted therapy; specifically, stomatitis grade II in two patients
and headache grade II in one patient where discontinuation of therapy was
unnecessary. Only in one patient treated with palovarotene, a cutaneous toxicity
grade III, was the cause of treatment discontinuation.
Treatment groups were homogeneous as no significant differences in sex, age, molecular status, and concomitant treatments were found. Treatment details for both groups are reported in Table 3.
Table 3.
(A) Target therapy DIPG patients: first-line and relapse treatment details. (B) Control group DIPG patients: first-line and relapse treatment details.
(A)
Patients | Age at diagnosis (years) | Gender | Backbone treatment | Target treatment | Target finding | Other treatment | Sides effects | OS (months) |
---|---|---|---|---|---|---|---|---|
1 | 11.93 | M | RTX![]() ![]() | Everolimus | m/pmTOR IHC expression | bevacizumab N/V re-irradiation | Aftosis stomatitis grade II | 26.57 |
2 | 5.06 | F | RTX![]() ![]() | Palovarotene | ACVR1 mutation | Bevacizumab temozolomide re-irradiation | No | 14.37 |
3 | 8.77 | F | RTX![]() ![]() | Pazopanib | PDGFRA | Bevacizumab temozolomide re-irradiation | Headache grade II | 18.00 |
4 | 5.04 | F | RTX![]() ![]() | Everolimus | m/pmTOR IHC expression | Bevacizumab temozolomide | No | 13.97 |
5 | 5.65 | F | RTX![]() ![]() | everolimus | m/pmTOR IHC expression | No (refused) | No | 12.07 |
6 | 5.98 | M | RTX![]() ![]() | everolimus | m/pmTOR IHC expression | Bevacizumab temozolomide re-irradiation | No | 20.47 |
7 | 6.61 | M | RTX![]() ![]() | everolimus and vemurafenib | m/pmTOR and BRAF IHC expression | Bevacizumab temozolomide re-irradiation | Aftosis stomatitis grade II | 27.50 |
8 | 7.79 | F | RTX![]() ![]() | everolimus | m/pmTOR IHC expression | Bevacizumab temozolomide re-irradiation | No | 32.20 |
9 | 6.01 | F | RTX![]() ![]() | palovarotene | ACVR1 mutation | Bevacizumab temozolomide re-irradiation | Grade III skin toxicity | 20.43 |
(B)
Patients | Age at diagnosis (years) | Gender | Backbone treatment | Other treatment | OS (months) |
---|---|---|---|---|---|
10 | 4.06 | F | RTX![]() ![]() | No (refused) | 7.10 |
11 | 5.44 | M | RTX![]() ![]() | Bevacizumab temozolomide re-irradiation | 14.33 |
12 | 6.10 | M | RTX![]() ![]() | Bevacizumab temozolomide | 24.47 |
13 | 4.18 | F | RTX![]() ![]() | Bevacizumab temozolomide re-irradiation | 21.97 |
14 | 5.56 | M | RTX![]() ![]() | Bevacizumab temozolomide re-irradiation | 11.40 |
15 | 9.44 | F | RTX![]() ![]() | Bevacizumab N/V re-irradiation | 18.53 |
16 | 6.62 | F | RTX![]() ![]() | Bevacizumab temozolomide | 13.33 |
17 | 9.31 | F | RTX![]() ![]() | Bevacizumab temozolomide re-irradiation | 14.17 |
18 | 3.60 | F | RTX![]() ![]() | Bevacizumab temozolomide re-irradiation | 15.03 |
19 | 6.05 | M | RTX![]() ![]() | No (refused) | 7.43 |
20 | 6.66 | F | RTX![]() ![]() | Bevacizumab temozolomide re-irradiation | 21.87 |
21 | 5.58 | F | RTX![]() ![]() | No (refused) | 4.90 |
22 | 5.04 | F | RTX![]() ![]() | Bevacizumab temozolomide re-irradiation | 17.57 |
23 | 7.73 | M | RTX![]() ![]() | Bevacizumab temozolomide re-irradiation | 12.27 |
24 | 14.71 | M | RTX![]() ![]() | Bevacizumab temozolomide | 7.87 |
25 | 6.35 | F | RTX![]() ![]() | Bevacizumab temozolomide re-irradiation | 13.77 |
N, nimotuzumab; OS, overall survival; RTX, radiotherapy; V, vinorelbine.
Radiological response to targeted treatment
Response to treatment according to the most recent RAPNO criteria 25 included progression disease in 3/9, stable disease in 4/9, and partial response in 2/9, documented an overall response rate in the target treatment group of 66% (6/9). Clinical timeline of four selected patients (one for each targeted treatment regimen) including MRI at indicated time points is detailed in Figure 3. Interestingly, no difference was found comparing prospective response assessment (RANO) and retrospective blinded re-evaluation using RAPNO criteria. No correlation was found between IHC expression intensity of mTOR/p-mTOR and radiological response in patients treated with everolimus.
Survival
The 2-year OS was 19.8% for the whole study population. Specifically, the median
OS time was longer in the targeted treatment group, reaching 20.26months
[interquartile range (IQR): 14.21–27.25] versus 14.18
months
(IQR: 11.76–19.19) for the non-targeted treatment group (Figure 4), p
=
0.032.
The longest survival time (32.2
months) was observed in one patient treated with
everolimus. Median SFP was 10.73
months (IQR: 6.88–14.54) in the targeted
treatment cohort and 7.77 (IQR: 4.65–10.21) in the non-targeted treatment group
(Figure 5),
log-rank test p
=
0.23.
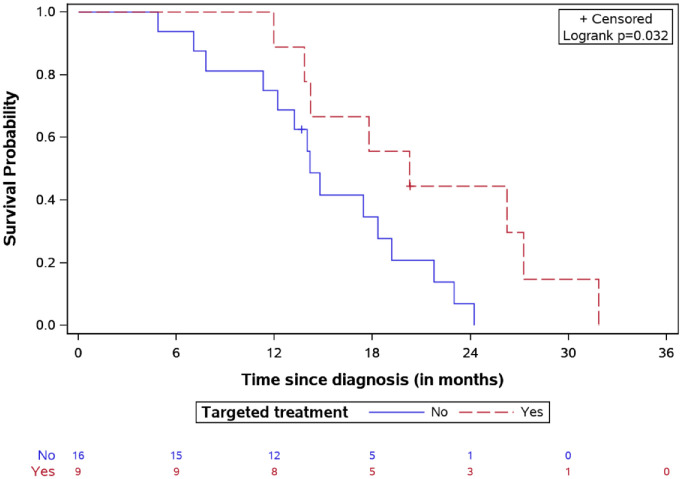
OS for DIPG patients treated by targeted agents versus classic treatment with number of patients at risk.
DIPG, diffuse intrinsic pontine glioma; OS, overall survival.
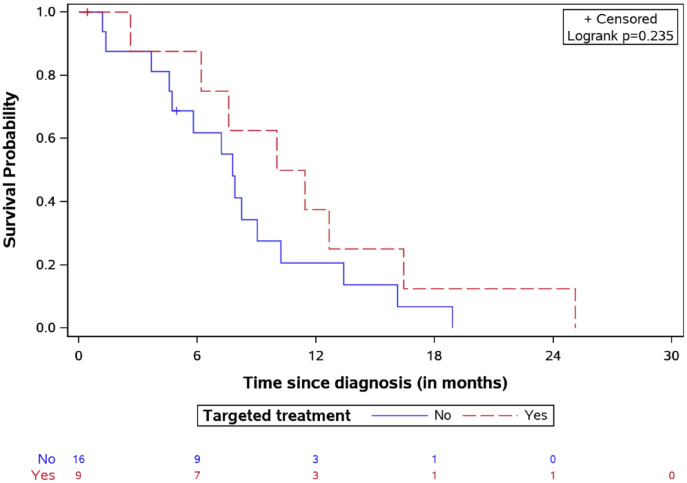
Survival after first relapse for DIPG patients treated by targeted agents versus classic treatment with number of patients at risk.
DIPG, diffuse intrinsic pontine glioma.
The median TTP after starting targeted therapies was 5.3months (range:
1.13–18.73).
Univariate analysis showed that the median OS in ACVR1-mutated
cases was 19.3months versus 13.9
months for wild-type
(log-rank test p
=
0.14). The K27M mutation in the H3.3 histone
was associated with a significantly shorter survival compared to the H3.1 (OS
13.9 versus 20.3, respectively, log-rank test
p
=
0.03, Figure 6). Patients with lower p53 expression (<50% in IHC) had a
better survival, with a median OS of 20.03
months versus
13.11
months for patients with higher expression (log-rank test
p
=
0.02, Figure 7).
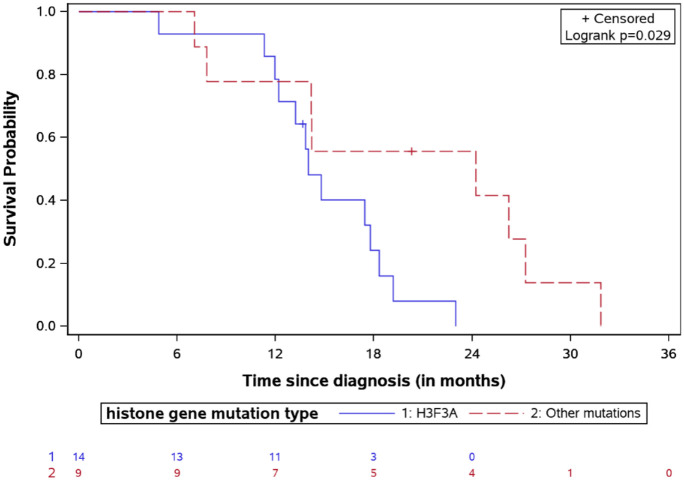
OS for DIPG patients stratified by histone mutation type with the number of patients at risk.
DIPG, diffuse intrinsic pontine glioma; OS, overall survival.
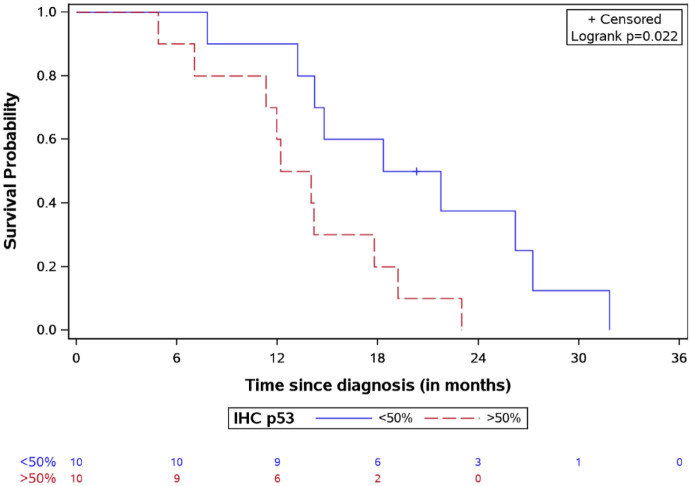
OS for DIPG patients stratified by IHC p53 expression with the number of patients at risk.
DIPG, diffuse intrinsic pontine glioma; IHC, immunohistochemistry; OS, overall survival.
Notably, the only patient refusing second-line treatment, except everolimus as a
single agent, had the shortest OS (12.07months) among the targeted therapy
group (Table
3A).
Discussion
Despite the considerable progress achieved in the treatment of pediatric brain
tumors, DIPG still remains a universally fatal disease with a median OS of 8–11months.
3
DIPG is standardly diagnosed using radiological imaging. Stereotactic needle biopsy is still more commonly recommended for cases with atypical radiological features or used within clinical trials which require the knowledge of the molecular alterations for patient enrollment.15,16
However, autopsy and more recently biopsy have largely contributed to advance our understanding on DIPG, unveiling a biologically complex pediatric brain cancer. Beyond histology, molecular and epigenetic dysregulation outline that each tumor is slightly different from others.
This aspect potentially contributes to the substantial failure of all therapeutic approaches attempted so far.
The urgent need to improve OS rate of patients with this devastating tumor has led to reconsidering the role of biopsy, to confirm the radiological diagnosis and to collect biological samples to perform molecular investigations. Due to modern surgical techniques, the operative risk of brainstem biopsies has progressively decreased, and biopsy has been shown to be safe in experienced centers. 26
Since 2015, we routinely perform the stereotactic biopsy under approval of our local Ethics Committee. The combination of robot assistance and pre-coronary approach offers an optimal diagnostic yield with a favorable risk profile, which we have already reported. 26 In line with our experience, other groups have shown that biopsy for DIPG patients contributes to a diagnostic detection rate ranging from 96% to 100% with a risk of mortality and morbidity lower than 5%. 35 As described by Puget et al. in a series of 130 DIPG patients, 36 biopsy was performed with a 100% diagnostic tissue sample obtained in all patients of our cohort at diagnosis or at disease progression. We did not record severe neurological deficits related to procedure, this finding confirming safety of the procedure. Previous recommendations limiting biopsy in DIPG patients to clinical trial settings 37 might not reflect the favorable safety profile that has emerged from more recent evidence. 38
The main aim of our study was to compare the OS and SFP in two cohorts of patients with radiological and molecularly confirmed diagnosis of DIPG, treated with either our standard institutional treatment or with a targeted treatment.
Upon diagnosis at our center, 23/25 patients received first-line backbone treatment with focal irradiation associated with nimotuzumab and vinorelbine 15 ; 2/25 patients, prior to admission to our hospital, had received focal RT with temozolomide.
At disease progression, 23/25 patients received second-line treatment with
bevacizumab and temozolomide, while second-line treatment with nimotuzumab and
vinorelbine was reserved for the two patients who received temozolomide as
first-line treatment. Re-irradiation was reserved, according to the literature
available, to those patients in whom 6months have relapsed since the first irradiation.
31
Based on the molecular data and after discussion with the molecular tumor board, at the TTP, targeted therapy was given to nine patients, in association with the backbone of the second-line treatment, never during RT, neither to patients with advanced stage of disease.
We detected targetable alterations in 15/25 patients (60%), including ACVR1, mTOR/p-mTOR, BRAFv600E, and PDGFRA.
We detected a positivity to mTOR by IHC in 70% of our cases, a finding in line with
previously reported data
39
; our study confirms that the PI3K/AKT/mTOR signaling is the most frequently
aberrant pathway in DIPG. mTOR is a serine/threonine kinase protein that has been
shown to control cell metabolism, cell survival, and cytoskeletal organization.
40
Several clinical trials have taken advantage of such target using rapamycin
or an analog drug, showing antitumor activity and mild toxicity in patients
41
even in the context of DIPG.42,43 At the time of disease
progression, 5/10 patients with positive immunostaining for mTOR/p-mTOR pathway
received everolimus in association to second-line therapy. Three patients, at the
time of detection of the targetable lesions, had an advanced stage of disease and
one was treated with palovarotene due to the ACVR1 mutation. The
only adverse event we reported in the everolimus cohort of patients was stomatitis
and headache in one patient, without needing treatment discontinuation. The median
OS described in the everolimus cohort was 20.47months, including the case with the
longest observed OS (32.2
months). The median SFP was 11.45
months. No correlation
was found between intensity of expression of mTOR/p-mTOR, as previously described in
low-grade gliomas.
42
BRAFv600E mutation was detected in one case which also presented
mTOR/p-mTOR pathway alteration. The gene BRAF encodes for a serine-threonine kinase
that drives cell growth. Although it represents the main oncogenic feature among
low-grade gliomas, BRAFv600E mutations are also found in about 6%
of pediatric high-grade gliomas,
28
and the coexistence of H3K27M and BRAFv600E mutations is
reported in several cases of pediatric gliomas.
43
Our patient received a combined treatment with vemurafenib and everolimus,
achieving 27.5months of OS and an SFP of 16.42
months. Our patient developed grade
II stomatitis, which is described as potential side effect following treatment with
everolimus.
Recurrent activating mutations in ACVR1 gene have been reported in
21% of DIPG patients.
18
In the ACVR1-mutated population, a marked female
predominance and a longer OS compared to DIPG patients with wild-type
ACVR1 (median 14.9months versus 10.9
months)
has been reported.
18
In our study cohort, ACVR1 mutations were found in 33.3% of
patients. Gain-of-function mutations in the Type I Bone Morphogenic Protein (BMP)
receptor ACVR1 have been identified in Fibrodysplasia Ossificans
Progressiva (FOP) as a germline mutation. The role of somatic ACVR1
mutation in DIPG remains under investigation.
44
Palovarotene is a retinoic receptor agonist active against BMP signaling.
Previous reports in patients with FOP showed some benefit upon treatment with palovarotene.
45
Gojo et al.
34
described the first case of DIPG harboring a ACVR1 mutation
treated by palovarotene, resulting in disease stabilization lasting 30
weeks and
providing first evidence of this approach for H3K27M-ACVR1 concurrently mutated
tumors. In our targeted therapy group, palovarotene was used for two patients. The
OS reported was 14.3 and 20.4
months, and SFP was 6.2 and 13.5
months, respectively.
Cutaneous toxicity is the most common side effect of palovarotene,
46
and a toxicity grade III was the cause of treatment discontinuation for one
of our patients.
Due to the small size of the tissue, NGS was performed globally in 14 patients, and
PDGFRA mutation was detected in one case.
PDGFRA can be targeted using tyrosine-kinase inhibitors (TKIs).
39
Specifically, pazopanib is a second-generation multi-targeted TKI against
VEGFR-1, 2 and 3, PDGFRA,
PDGFR-beta, and c-kit,47,48 which has
shown activity and good tolerability in patients with advanced renal cell carcinoma
and soft-tissue sarcomas.49,50 In our case, the patient was treated with pazopanib after first
progression of the disease. The OS was 18months and SFP 7.58
months. During
treatment, grade II headache was detected.
As mentioned, 9/25 patients were treated with a targeted therapy. Comparing treatment
and non-targeted treatment group, the median OS in the group treated with targeted
agents was significantly different with 20.26months (IQR: 14.21–27.25)
versus 14.18
months (IQR: 11.76–19.19) in the control group.
The longest OS registered was 32.2
months in a patient with mTOR/p-mTOR
overexpression and treated with everolimus. Moreover, the median SFP time was
10.73
months (IQR: 6.88–14.54) in the targeted treatment cohort and 7.77 (IQR:
4.65–10.21) in the control group. Only 1/9 patients in the target group treated with
palovarotene experienced a grade III toxicity, which led to the suspension of
treatment. No toxicity was registered in the non-targeted treatment group. No severe
adverse events were reported in both groups.
In our experience, everolimus was the treatment that conferred the best OS. Notably, the only patient received everolimus as a single agent had the worst OS, suggesting the advantage of a combined therapeutic approach.
The only previous series described in literature that was treated with targeted
agents, either at diagnosis or at disease progression and received backbone therapy
including focal irradiation was published by Gojo et al.,
34
who reported the data of nine patients with H3K27M mutant diffuse midline
glioma which included seven DIPGs. Targeted therapy included inhibition of the
PI3K/AKT/mTOR pathway, MAPK signaling, immunotherapy, receptor tyrosine kinase
inhibition, and retinoic receptor agonist. The overall response rate described was
78% including one complete remission, three partial responses, and three stable
diseases; median TTP was 29weeks. In our targeted therapy cohort, the response rate
was 66% (four stable disease and two partial response). The different response in
our study compared to Gojo et al. may be related to our selected
population that include only DIPG and not DMG patients. Conversely, median OS
described by Gojo et al. in target population was shorter than that
of our patients (16.5
months versus 20.26
months).
34
The decision to treat patients on the basis of target detection tout court might be a limitation of our study. Development of an integrated data platform with bioinformatics tools has been advocated to guide precision medicine approaches and standardize treatment response assessment. 51
Even though re-irradiation has been shown to improve OS, it was equally represented in our study groups, suggesting a negligible role in determining the outcome differences we observed.
To the best of our knowledge, this is the first study reporting on a DIPG population treated by targeted therapies in comparison to the standard treatment currently utilized in our institution. Despite the limitations of the study in terms of the small sample size and the retrospective design, we find our results to be promising. These results showed an improvement in OS and SFP. A strong re-consideration of tumor biopsy as routine practice in patients with DIPG diagnosis is required. If practiced in experienced centers, biopsy would certainly allow for the detection of molecular alterations and the use of more targeted therapies. Based on our preliminary data that suggest prognostic advantage and the relatively low risk of severe side effects, we strongly advocate to reconsider performing a biopsy on all DIPG patients at diagnosis or at progression, according to the patient’s clinical conditions. This allows for the detection of potential molecular driver alterations that can be targetable and subsequently adding targeted drugs to first-line treatment with the intent to improve the OS rate of this severe disease.
Supplemental Material
Supplemental material, sj-docx-1-tam-10.1177_17588359221113693 for Targeted therapy for pediatric diffuse intrinsic pontine glioma: a single-center experience by Giada Del Baldo, Andrea Carai, Rachid Abbas, Antonella Cacchione, Mara Vinci, Valentina Di Ruscio, Giovanna Stefania Colafati, Sabrina Rossi, Francesca Diomedi Camassei, Nicola Maestro, Sara Temelso, Giulia Pericoli, Emmanuel De Billy, Isabella Giovannoni, Alessia Carboni, Martina Rinelli, Emanuele Agolini, Alan Mackay, Chris Jones, Silvia Chiesa, Mario Balducci, Franco Locatelli and Angela Mastronuzzi in Therapeutic Advances in Medical Oncology
Supplemental material, sj-docx-2-tam-10.1177_17588359221113693 for Targeted therapy for pediatric diffuse intrinsic pontine glioma: a single-center experience by Giada Del Baldo, Andrea Carai, Rachid Abbas, Antonella Cacchione, Mara Vinci, Valentina Di Ruscio, Giovanna Stefania Colafati, Sabrina Rossi, Francesca Diomedi Camassei, Nicola Maestro, Sara Temelso, Giulia Pericoli, Emmanuel De Billy, Isabella Giovannoni, Alessia Carboni, Martina Rinelli, Emanuele Agolini, Alan Mackay, Chris Jones, Silvia Chiesa, Mario Balducci, Franco Locatelli and Angela Mastronuzzi in Therapeutic Advances in Medical Oncology
Acknowledgments
The authors thank Megan Eckley for helping in the English final version.
Footnotes
Supplemental material: Supplemental material for this article is available online.
Contributor Information
Giada Del Baldo, Department of Pediatric Haematology and Oncology, and Cell and Gene Therapy, Bambino Gesù Children’s Hospital, IRCCS, Rome, Italy.
Andrea Carai, Neurosurgery Unit, Department of Neurosciences, Bambino Gesù Children’s Hospital, IRCCS, Piazza Sant’Onofrio 4, 00165 Rome, Italy.
Rachid Abbas, CESP, INSERM, Université Paris Sud, Villejuif, France.
Antonella Cacchione, Department of Pediatric Haematology and Oncology, and Cell and Gene Therapy, Bambino Gesù Children’s Hospital, IRCCS, Rome, Italy.
Mara Vinci, Department of Pediatric Haematology and Oncology, and Cell and Gene Therapy, Bambino Gesù Children’s Hospital, IRCCS, Rome, Italy.
Valentina Di Ruscio, Department of Pediatric Haematology and Oncology, and Cell and Gene Therapy, Bambino Gesù Children’s Hospital, IRCCS, Rome, Italy.
Giovanna Stefania Colafati, Oncological Neuroradiology Unit, Imaging Department, Bambino Gesù Children’s Hospital, IRCCS, Rome, Italy.
Sabrina Rossi, Pathology Unit, Department of Laboratories, Bambino Gesù Children’s Hospital, IRCCS, Rome, Italy.
Francesca Diomedi Camassei, Pathology Unit, Department of Laboratories, Bambino Gesù Children’s Hospital, IRCCS, Rome, Italy.
Nicola Maestro, Department of Pediatric Haematology and Oncology, and Cell and Gene Therapy, Bambino Gesù Children’s Hospital, IRCCS, Rome, Italy.
Sara Temelso, Division of Molecular Pathology, The Institute of Cancer Research, London, UK. Division of Cancer Therapeutics, The Institute of Cancer Research, London, UK.
Giulia Pericoli, Department of Pediatric Haematology and Oncology, and Cell and Gene Therapy, Bambino Gesù Children’s Hospital, IRCCS, Rome, Italy.
Emmanuel De Billy, Department of Pediatric Haematology and Oncology, and Cell and Gene Therapy, Bambino Gesù Children’s Hospital, IRCCS, Rome, Italy.
Isabella Giovannoni, Pathology Unit, Department of Laboratories, Bambino Gesù Children’s Hospital, IRCCS, Rome, Italy.
Alessia Carboni, Oncological Neuroradiology Unit, Imaging Department, Bambino Gesù Children’s Hospital, IRCCS, Rome, Italy.
Martina Rinelli, Laboratory of Medical Genetics, Bambino Gesù Children’s Hospital, IRCCS, Rome, Italy.
Emanuele Agolini, Laboratory of Medical Genetics, Bambino Gesù Children’s Hospital, IRCCS, Rome, Italy.
Alan Mackay, Division of Molecular Pathology, The Institute of Cancer Research, London, UK. Division of Cancer Therapeutics, The Institute of Cancer Research, London, UK.
Chris Jones, Division of Molecular Pathology, The Institute of Cancer Research, London, UK. Division of Cancer Therapeutics, The Institute of Cancer Research, London, UK.
Silvia Chiesa, Department of Radiotherapy, Fondazione Policlinico Universitario “A. Gemelli,” Catholic University of Sacred Heart, Rome, Italy.
Mario Balducci, Department of Radiotherapy, Fondazione Policlinico Universitario “A. Gemelli,” Catholic University of Sacred Heart, Rome, Italy.
Franco Locatelli, Department of Pediatric Haematology and Oncology, and Cell and Gene Therapy, Bambino Gesù Children’s Hospital, IRCCS, Rome, Italy. Department of Life Sciences and Public Health, Fondazione Policlinico Universitario “A. Gemelli,” Catholic University of Sacred Heart, Rome, Italy.
Angela Mastronuzzi, Department of Pediatric Haematology and Oncology, and Cell and Gene Therapy, Bambino Gesù Children’s Hospital, IRCCS, Rome, Italy.
Declarations
Ethics approval and consent to participate: All targeted drugs were given for compassionate use and Ethics Committee approval was obtained for every case. Biological samples were collected in accordance with the Ethics Committee of the Bambino Gesù Children’s Hospital (Ethics Committee Approvals N°1343/2018, N°969/2015, N°1952/2019) and with the 1964 Helsinki Declaration which includes its later amendments or comparable ethical standard; written informed consent was obtained by patient’s legal guardian.
Consent for publication: Not applicable.
Author contribution(s): Giada Del Baldo: Conceptualization; Data curation; and Writing – original draft.
Andrea Carai: Conceptualization; Methodology; and Writing – original draft.Rachid Abbas: Data curation; Formal analysis; and Software.
Antonella Cacchione: Data curation and Visualization.
Mara Vinci: Data curation; Investigation; and Visualization.
Valentina Di Ruscio: Data curation.
Giovanna Stefania Colafati: Data curation.
Sabrina Rossi: Data curation; Investigation.
Francesca Diomedi Camassei: Investigation.
Nicola Maestro: Investigation.
Sara Temelso: Investigation.
Giulia Pericoli: Investigation.
Emmanuel De Billy: Investigation.
Isabella Giovannoni: Investigation.
Alessia Carboni: Data curation.
Martina Rinelli: Investigation.
Emanuele Agolini: Investigation.
Alan Mackay: Investigation.
Chris Jones: Investigation.
Silvia Chiesa: Data curation.
Mario Balducci: Data curation.
Franco Locatelli: Conceptualization; Supervision; and Writing – review & editing.
Angela Mastronuzzi: Conceptualization; Supervision; and Writing – review & editing.
Funding: The authors disclosed receipt of the following financial support for the research, authorship, and/or publication of this article: This work was funded by Italian Ministry of Health Ricerca Corrente, DIPG Italia and Il coraggio dei bambini Onlus. MV is a CwCUK Fellow.
Competing Interests: The authors declare that there is no conflict of interest.
Availability of data and materials: Not applicable.
References
Articles from Therapeutic Advances in Medical Oncology are provided here courtesy of SAGE Publications
Full text links
Read article at publisher's site: https://doi.org/10.1177/17588359221113693
Read article for free, from open access legal sources, via Unpaywall:
https://journals.sagepub.com/doi/pdf/10.1177/17588359221113693
Citations & impact
Impact metrics
Citations of article over time
Alternative metrics

Discover the attention surrounding your research
https://www.altmetric.com/details/136009453
Article citations
Towards consistency in pediatric brain tumor measurements: Challenges, solutions, and the role of artificial intelligence-based segmentation.
Neuro Oncol, 26(9):1557-1571, 01 Sep 2024
Cited by: 1 article | PMID: 38769022
Review
Molecular Characterization and Treatment Approaches for Pediatric H3 K27-Altered Diffuse Midline Glioma: Integrated Systematic Review of Individual Clinical Trial Participant Data.
Cancers (Basel), 15(13):3478, 03 Jul 2023
Cited by: 2 articles | PMID: 37444588 | PMCID: PMC10340772
Review Free full text in Europe PMC
Bench-to-bedside investigations of H3 K27-altered diffuse midline glioma: drug targets and potential pharmacotherapies.
Expert Opin Ther Targets, 27(11):1071-1086, 01 Jul 2023
Cited by: 2 articles | PMID: 37897190
Review
Paediatric-type diffuse high-grade gliomas in the 5th CNS WHO Classification.
Pathologica, 114(6):422-435, 01 Dec 2022
Cited by: 20 articles | PMID: 36534421 | PMCID: PMC9763979
Review Free full text in Europe PMC
Pediatric diffuse midline glioma H3K27- altered: A complex clinical and biological landscape behind a neatly defined tumor type.
Front Oncol, 12:1082062, 16 Jan 2023
Cited by: 15 articles | PMID: 36727064 | PMCID: PMC9885151
Review Free full text in Europe PMC
Go to all (6) article citations
Similar Articles
To arrive at the top five similar articles we use a word-weighted algorithm to compare words from the Title and Abstract of each citation.
Nimotuzumab-vinorelbine combination therapy versus other regimens in the treatment of pediatric diffuse intrinsic pontine glioma.
Childs Nerv Syst, 40(6):1671-1680, 13 Mar 2024
Cited by: 0 articles | PMID: 38478066
Survival benefit for patients with diffuse intrinsic pontine glioma (DIPG) undergoing re-irradiation at first progression: A matched-cohort analysis on behalf of the SIOP-E-HGG/DIPG working group.
Eur J Cancer, 73:38-47, 03 Feb 2017
Cited by: 62 articles | PMID: 28161497
Nimotuzumab-containing regimen for pediatric diffuse intrinsic pontine gliomas: a retrospective multicenter study and review of the literature.
Childs Nerv Syst, 35(1):83-89, 11 Nov 2018
Cited by: 5 articles | PMID: 30417211
Pediatric diffuse intrinsic pontine glioma: where do we stand?
Cancer Metastasis Rev, 38(4):759-770, 01 Dec 2019
Cited by: 28 articles | PMID: 31802357
Review
Funding
Funders who supported this work.