Abstract
Free full text

Gap junction and hemichannel-independent actions of connexins on cell and tissue functions – An update
Abstract
Connexins, a family of transmembrane proteins, are components of both gap junction channels and hemichannels, which mediate the exchange of ions and small molecules between adjacent cells, and between the inside and outside of the cell, respectively. Substantial advancements have been made in the comprehension of the role of gap junctions and hemichannels in coordinating cellular events. In recent years, a plethora of studies demonstrate a role of connexin proteins in the regulation of tissue homeostasis that occurs independently of their channel activities. This is shown in the context of cell growth, adhesion, migration, apoptosis, and signaling. The major mechanisms of these channel-independent activities still remain to be discovered. In this review, we provide an updated overview on the current knowledge of gap junction- and hemichannel-independent functions of connexins, in particular, their effects on tumorigenesis, neurogenesis and disease development.
1. Introduction
Connexins are proteins which form both gap junctions and hemichannels, which are ubiquitously found in both vertebrates and invertebrates. Unapposed connexin hemichannels, which are composed of six connexin molecules oligomerized into a cylinder, are found at the plasma membrane, where they allow the exchange of small molecules (<1.2 kD) between the intracellular and extracellular environments. As a newcomer of connexin-forming channels for over a decade, hemichannels are implicated in cellular responses to various physiological conditions and metabolic and oxidative stresses [1]. Gap junctions are hexameric channels formed by two docked hemichannels from adjacent cells, which allow the transfer of small molecules between the adjoining cells. Many physiological processes such as cell growth and cell death are driven by the substances that are transferred through these channels [2]. These molecules that pass through gap junctions include ions, second messengers, and small metabolites. Thus, gap junctional intercellular communication (GJIC) is essential for the maintenance and regulation of cell differentiation, tissue physiology, and the normal functions of organs [3,4]. Since the molecules that cross through hemichannels are similar to those which travel through GJIC, hemichannel-dependent signaling is also seen as a significant mediator of tissue homeostasis. Thus far, there are 20 different types of connexins which are known to exist in the mouse and 21 types in humans, and the permeability and signaling properties of the individual gap junctions and hemichannels are defined by their specific connexins. It is well documented that GJIC is important in mediating normal cell growth, differentiation, and development. Non-coupling or non-communicating gap junctions and hemichannels result in the disruption of normal homeostasis. Among the various types of connexins, mutations or loss of functional channels are implicated in many diseases and disorders such as congenital deafness, skin disorders, cataracts and cancers [4,5]. Intriguingly, some disease-causing mutants of connexins form functional channels [4] as those formed by wild-type connexins, which implies channel-independent roles of connexins.
In recent years, more studies have focused on the gap junction-and hemichannel-independent roles of connexins. Connexins are reportedly able to influence cell adhesion, migration, and cell cycle in a GJIC-independent manner [6–9]. A plethora of connexin-associated proteins have been discovered, including cytoskeletal elements, enzymes, adhesion molecules, and signaling molecules. These connexin-associated proteins are shown to regulate a number of mechanisms involved in both channel-dependent and independent functions by connexins [8–10]. Additionally, GJIC independent involvement of connexin-associated intercellular adhesion has also been presented [7]. We previously provided an overview of the gap junction-independent functions of connexins on cell growth, differentiation and tumorigenicity [6]. Here, we provide an updated summary on the growing list of channel-independent functions of connexins. These include the role of connexins in cell growth, migration, apoptosis, signaling and development. Furthermore, we discuss the impact of dysregulated connexins on tumorigenesis and disease development.
2. Gap junction-independent functions of connexins on tumorigenic cell growth, migration, and apoptosis
Loewenstein and Kanno [11] first established evidence that liver cancer cells were different from normal liver cells in that they lacked intercellular communication. Since then, there has been a surge of studies dedicated to discovering the role of gap junctions and connexins in tumor progression [5,12]. Reduced expression of connexins and GJIC has been shown in many tumor types, and connexins have been recognized as tumor suppressors. Clinical studies show that deficient or abnormal connexins are frequently found in tumor tissues and cell lines, such as breast cancer, prostate cancer, lung cancer, and many other cancers. Connexins are considered to be tumor suppressors through the transmission of signaling molecules that regulate cell proliferation, migration, and apoptosis. However, recently, more studies acknowledge that these effects on cancer behavior may depend on the connexin protein itself, rather than on the cell–cell coupling [13]. Although there remains experimental evidence regarding the involvement of gap junctions in controlling cell growth, migration, and tumorigenesis, there is mounting evidence supporting the position of GJIC-independent roles of connexins in cancer cell growth and progression (see Table 1 for a summary).
Table 1
Summary of the published studies of gap junction-independent functions of connexins on tumorigenic cell growth, migration, apoptosis, and cell signaling.
References | Cell | Connexin | GJIC-independent functions |
---|---|---|---|
Kalra et al. [14] | MDA-MB-435 cells | Cx26 | Inhibits anchorage-independent growth and cell migration. Decreases β1 integrin and MMP-9 activity. Increases TIMP-1 activity |
Xu et al. [15] | NSCLC patient samples and LH7 cells | Cx43 | Inhibits proliferation and cell cycle transition |
Ionta et al. [16] | HTC and BRL3A cells | Cx43 | Decreases cell growth and reverts the transformed phenotype |
Li et al. [17] | HuH7 and Li-7 cells | Cx32 | Increases cell proliferation, migration, and invasion |
Lamiche et al. [18] | LNCaP and PC3 cells | Cx43 | Increases migration in LNCaP cells Decreases proliferation, adhesion, and invasion in PC3 cells |
Zucker et al. [19] | WM793B and 1205Lu cells | Cx43 | Decreases anchorage-independent growth and increases invasion |
Zhang et al. [20] | BEAS-2B, WI-38, Calu-3, A549, NCI-H1299, NCI-H460, and NCI-H226 cells | Cx31.1 | Decreases cell proliferation, delays cell cycle at G1 phase, decreases migration and invasion |
McLachlan et al. [21] | MDA-MB-231 and MDA-MB-435 cells | Cx26 Cx43 | Decreases anchorage-independent growth and migration. Reverses EMT Inhibits endothelial cell tubulogenesis and migration, reducing tumor angiogenesis |
Fukushima et al. [22] | PC3 and LNCaP cells | Cx43 | In combination with Docetaxel, inhibits cell growth and induces apoptosis |
Mauro et al. [23] | JKT-1 cells | Cx43 | In combination with Griseofulvin, induces apoptosis |
Sun et al. [24] | BxPC-3, SW1990, PaTu8988, PANC-1, AsPC-1 and CFPAC-1 cells | Cx43 | Increase apoptosis and inhibit cell growth |
Sato et al. [25] | A549 cells | Cx32 | In combination with Vinorelbine, induces apoptosis and decreases cell proliferation |
Fujimoto et al. [32] | Caki-1 cells | Cx32 | Induces apoptosis and decreases cell proliferation |
Langlois et al. [34] | REK, Hs 456.Sk, and SCC-13 cells | Cx43 | Cells with reduced Cx43 expression have increased EMT and invasive features |
Herrero-Gonzalez et al. [35] | C6 cells | Cx43 | Inhibits progression to S phase in the cell cycle, decreases rate of proliferation. Diminishes c-Src activity |
Crespin et al. [37] | LN18 and HEK293 cells | Cx43 | Inhibits cell growth and increases cell migration, and induces actin cytoskeleton reorganization by C-terminal tail of Cx43 |
Gellhaus et al. [38] | 293T cells | Cx43 | The C-terminal tail of Cx43 interacts with CCN3 and reduces the growth of 293T cells |
Johnstone et al. [39] | HeLa and HFF cells | Cx43 | Increased Cx43 expression delays mitotic durations and reduces cell proliferation |
Many studies suggest the significant inhibitory influence of connexins on the control of tumor cell growth and migration of many cancer cell types through a non-GJIC route. These effects were observed with Cx26 in breast cancer [14]. The role of Cx26 in MDA-MB-435 breast cancer migration and invasion was revealed by using three Cx26 mutants: one expressing GJIC-competent Cx26, a GJIC-incompetent Cx26, and a Golgi apparatus-localized disease- linked Cx26 mutant. This method was used to determine whether the expression of functional and GJIC-incompetent subtypes of Cx26 could change the tumorigenic characteristics of these cells. All three variants inhibited anchorage-independent growth and significantly reduced cell migration. Furthermore, expression of all Cx26 variants exhibited decreased β1 integrin level and MMP-9 activity, and increased TIMP-1 activity. This data suggests that Cx26 expression is independent of GJIC communication in reverting the tumorigenic properties of MDA-MB-435 cells. In lung cancer, Xu et al. [15] demonstrated the reduction of both Cx43 and E-cadherin expression in advanced non-small cell lung cancer (NSCLC) patient samples. After overexpressing Cx43 in the lung cancer cell line LH7, E-cadherin expression was found to be concurrently upregulated. Additionally, Cx43 overexpression was correlated with the inhibition of proliferation and cell cycle transition. This effect appears to be independent of GJIC, as the Cx43 was localized in the cytosol rather than at the cell surface. These cell proliferation inhibitory effects of Cx43 are also shown to be significant in the progression of liver cancer. Ionta et al. [16] found that the introduction of exogenous Cx43 expression into rat hepatocellular carcinoma (HCC) cells causes a decrease in cell growth as well as a reversion of the transformed phenotype. These effects were independent of GJIC, as there was no evidence of a difference in GJIC capacity when comparing the transfected cells versus control cells as assessed by dye transfer with Lucifer Yellow. However, there were changes in the phosphorylation pattern of Cx43, which could affect its ability to oligomerize and transport to the plasma membrane. These studies demonstrate how various connexins may impede the growth and migration of breast, lung, and liver cancer cells.
Several studies have shown that connexins are involved differently at distinct stages of tumor progression. At the primary tumor level, many connexins exhibit decreased expression or impaired trafficking, as we discussed in the previous examples. However, as the neoplastic lesions progress to more invasive and undifferentiated stages, the connexins can become upregulated [5]. In HCC, increased Cx32 protein enhanced its progression through increased invasion and metastasis, despite being localized in the cytoplasm [17]. By utilizing an inducible expression system of Cx32 in a human HCC cell line, it was discovered that despite a dramatic increase in Cx32 expression, none of the cells were coupled by the dye, and Cx32 protein was retained primarily in the Golgi apparatus. Additionally, the induced expression of Cx32 caused a significant increase in proliferation rate as well as migration and invasion. Furthermore, an in vivo model demonstrated increased metastatic lesions in mice with induced Cx32 expression cells. A report by Lamiche et al. [18] showed that the localization and functionality of Cx43 in prostate cancer cells may govern its ability to metastasize to bone. Two different prostate cancer cell lines were used. In LNCaP cell lines, Cx43 was functional and localized at the plasma membrane, which allowed the cells to become highly aggressive as well as creating more osteolytic metastases in bone xenografts. In PC3 cells, Cx43 was localized in the cytoplasm and nonfunctional, leading to a reduction in proliferation, adhesion, and invasion. Thus, the differential effects of Cx43 channels on prostate cancer cells are likely to be primarily caused by the types of prostate cancer cells used. The dual role of connexins in cancer behavior is also investigated in metastatic melanoma behavior, where Zucker et al. [19] examined the effects of a third transmembrane domain mutation (T154A) in Cx43, resulting in a dominant negative Cx43 mutant in which the channels remained closed. The dye preloading assay with calcein-AM and DiI demonstrated that pre-metastatic WM793B and metastatic 1205Lu melanoma cells transfected with the T154A Cx43 mutant were deficient in channel coupling. Transfection of the metastatic 1205Lu melanoma cells with T154A reduced the anchorage-independent growth. Additionally, the 1205Lu–T154A Cx43 mutants exhibited increased invasion. These effects were not due to the lack of communication since the opposite effects were not observed with wild type Cx43. Despite these studies showing the stimulatory role of connexins on invasive cancers, other studies report otherwise. Zhang et al. [20] found that Cx31.1 is downregulated in five NSCLC cell lines as compared to normal human lung epithelial cells, and the expression is inversely related to the metastatic potential. After overexpressing Cx31.1 in the NSCLC cell line H1299, they found that Cx31.1 does not form functional gap junction channels and is localized intracellularly. Furthermore, the cell proliferation was decreased, cell cycle was delayed at the G1 phase, anchorage-independent growth was inhibited, and cell migration and invasion were suppressed. Additionally, xenografts of Cx31.1-overexpressing cells into nude mice had reduced tumorigenicity compared to control cells. They concluded that Cx31.1 may act as a tumor suppressor in NSCLC cell lines by reversing the epithelial to mesenchymal transition (EMT) process. These studies provide conflicting evidence of the precise role of connexins in regulating cancer growth and migration in a GJIC-independent fashion. Many of these studies suggest that the expression of connexins, but not the degree of channel formation, relates to tumor progression.
GJIC-independent connexin effects on tumorigenesis extend beyond proliferation and migration into other hallmarks of cancer, including angiogenesis and apoptosis. Cx26 and Cx43 further regulate other tumorigenic properties of MDA-MB-231 breast cancer cells, such as angiogenesis and EMT, in a GJIC-independent way [21]. The overexpression of either Cx26 or Cx43 reduced anchorage- independent growth and induced partial re-differentiation of MDA-MB-231 three-dimensional organoids. The connexins did not assemble into gap junctions at the plasma membrane, nor did they establish GJIC as assessed by dye transfer using fluorescence recovery after photobleaching (FRAP). It was also discovered that connexin overexpression caused a reduction in cell migration and promoted a reversal of EMT. Additionally, connexin expression could regulate the expression of molecules important in angiogenesis, such as monocyte chemotactic protein-1 (MCP-1) and IL-6. This study provided the first evidence of the role of connexins in regulating angiogenesis in breast tumors.
There is much evidence pointing to the additive effect of connexins in combination with other chemotherapeutic agents to regulate cancer apoptosis. The combination of Docetaxel (DTX) with the expression of the Cx43 gene was discovered to be the optimal treatment for advanced hormone refractory prostate cancer [22]. Transfection of the Cx43 gene by itself into PC3 cells did not inhibit tumor growth or increase GJIC assessed by calcein-AM and DiI dye transfer, but in combination with DTX, it inhibited cell growth and induced apoptosis. This was achieved through the downregulation of Bcl-2 expression and activation of the apoptosis pathway. The combinatorial use of Cx43 and DTX also significantly inhibited the growth of PC3 tumor xenografts. These results indicate that Cx43 is effective in enhancing the cytotoxicity of DTX by downregulating Bcl-2. Cx43 also aids in the apoptotic effects of germ cell tumors in response to Griseofulvin, an antifungal antimitotic drug, through translocation from the cytoplasm to the nucleus [23]. Cx43 immunoreactive signal was mainly found distributed throughout the cytoplasm of tumoral germ cells and there was no coupling found between adjacent cells. When viewed with three dimensional, high-resolution deconvolution microscopy, the cells treated with Griseofulvin had many Cx43 spots in the perinuclear region. Cx43 contains a nuclear targeting sequence in its C-terminal domain that is involved in the control of cell death gene expression, which explains why Cx43 is required for Griseofulvin to activate the caspase and apoptosis pathway. In pancreatic cancer, Cx43 is also shown to mediate apoptosis regulation [24]. Cx43 translocates to the mitochondria, where it interacts with Bax through the amino acid residues 241–382 and initiates the mitochondrial apoptotic pathway. When 18β-glycyrrhetinic acid (β-GA) was added to inhibit GJIC, Cx43 was still able to inhibit the growth and induce apoptosis in the BxPC-3 pancreatic cancer cells. Additionally, a dominant-negative Cx43 mutant (Cx43N) with deletion of amino acids 130–136, which inhibits Cx43-mediated GJIC, also preserved the tumor-suppressive activity. Cx32 is another connexin demonstrated to be effective in combinatorial therapy, as shown in lung adenocarcinoma, where it amplifies the cytotoxic effects of the chemotherapeutic drug Vinorelbine (VBN) [25]. This was shown to be partially due to GJIC effects, and partially GJIC-independent. When a gap junction inhibitor was added, there was a partial abrogation of VBN-induced cytotoxicity in A549 transfected with Cx32, but no effect was seen on control cells treated with VBN. These findings reveal the vital role of connexins in cancer progression and how they may be used in combination with chemotherapeutic drugs to prevent the advancement of various cancers. Many studies still report conflicting evidence regarding the exact role of connexins as either tumor suppressors or tumor promoters, and whether these actions are through the exchange of molecules through its gap junctions and hemichannels, or whether it is due to a GJIC-independent mechanism, especially in the more invasive and metastatic cancers. As seen in Fig. 1, evidence of the non-GJIC tumor suppressive effects of connexins seem to outweigh those reflecting the tumor promoter effects. Most notably, they demonstrate that connexins are a key player involved in inhibiting the progression of breast, prostate, and lung primary cancers. Furthermore, there are increasing confirmations from studies suggesting that many of the known connexin tumor suppressive effects are through GJIC-independent mechanisms [7–10,6].

Non-GJIC tumor promoting and tumor suppressing effects of connexins. The non-GJIC effects of connexins on tumor behavior can be separated into tumor promoter and tumor suppresser categories. These effects may further be subcategorized into influences on the tumor growth, migration, cell cycle, apoptosis, and EMT.
3. Gap junction-independent functions of connexins on tumorigenic cell cycle and signaling
Several studies from previous years have provided evidence of how connexin proteins influence cell growth independently of gap junction channel and hemichannel formation through its impact on cell cycle and signaling [10]. This is due to the influence of connexin proteins on the production and activity of many cell cycle regulators, such as p27Kip1, cyclin A, cyclin D1, cyclin D2, ERK1/2, Src, and FGF1, just to name a few [26–31]. For example, the C-terminal tail of Cx43 (243–382 aa), which lacks intrinsic gap junction activity, has been shown to be just as effective as the full protein in suppressing neuroblastoma progression through its effects on signaling molecules such as Src [8]. In a similar vein, Fujimoto et al. [32] demonstrate that the inactivation of Src increases the tumor suppressive effect of Cx32 in renal cancer cells. The authors previously demonstrated that Src is an important determinant in the malignancy of renal cell carcinoma [33]. In the current study, the combination of a Src inhibitor and Cx32 was effective in regulating the growth and survival by inducing a cytotoxic effect on renal cancer cells [32]. A more recent example of the influence of connexins on tumorigenic cell signaling and cell cycle is shown by Langlois et al., [34] who examined the effects of Cx43 interaction with the skin tumor suppressor caveolin-1 (Cav-1) in rat epidermal keratinocytes (REK). REK cells with reduced Cx43 expression exhibited increased EMT as well as more invasive features than the control cells. On the other hand, Cx43 overexpression caused an inhibition of the invasive properties. Blocking the gap junctions with carbenoxolone did not affect the invasive behavior; thus, GJIC was not involved. Furthermore, Cx43 was able to colocalize with Cav-1 through its C-terminal domain, and this interaction between the Cx43 C-terminal tail and Cav-1 played a crucial protective role in keratinocyte transformation and invasion (Fig. 2). This was also exemplified in vivo, where the colocalization of Cx43 and Cav-1 was reduced in human keratinocyte skin tumors such as epidermal squamous cell carcinoma cells, suggesting a protective role against keratinocyte transformation by the interaction between Cx43 and Cav-1. Further evidence of Cx43’s effects on cell cycle is exemplified in C6 glioma cells, where the restoration of Cx43 inhibits their progression to the S phase of the cell cycle, owing in part to a reduction of cyclin E expression, which decreases the rate of proliferation [35]. c-Src is known to phosphorylate Cx43 from previous studies [36], and interestingly, it is shown here that the restoration of Cx43 expression in glioma cells diminishes c-Src activity, exhibited by a decrease in the active form (Y416 c-Src) and an increase in the inactive form (Y527 c-Src). Additionally, a Cx43 mutant lacking its c-Src phosphorylation sites, Tyr247 and Tyr 265, was not able to inhibit the c-Src activity and did not exhibit decreased glioma proliferation. This suggests that the Tyr247 and Tyr 265 residues on the C-terminal tail of Cx43 are involved in the anti-proliferative effects (Fig. 2). This data suggests that Cx43 and c-Src are mutually regulated by a reciprocal activation/inactivation loop via protein phosphorylation. Connexins have also been implicated in the regulation of migration and proliferation through its interactions with the actin cytoskeleton. This study examined the effects of Cx43 in mediating the regulation of the actin cytoskeleton of human glioma cells [37]. The authors designed two truncated constructs of Cx43: one lacking the C-terminal tail and one lacking the entire transmembrane domain. It was discovered that there was a similar reduction in the proliferation of glioma cells regardless of whether the truncated versions or full length version of Cx43 were used. Truncated Cx43 does not alter GJIC, which was analyzed by dye transfer with calcein-AM. Additionally, the presence of a Cx43 C-terminal domain is sufficient to promote migration of glioma cells. The use of time-lapse imaging showed that the C-terminus is associated with a lamellipodia-type migration, suggesting that Cx43 signaling is involved in the regulation of the actin cytoskeleton. Gellhaus et al. [38] established a link between Cx43 and the growth regulator protein CCN3, which is involved in the anti-proliferative activity of neuroblastoma, glioma, and chronic myeloid leukemia. FRET analysis and co-immunoprecipitation were used to establish an interaction between CCN3 and the C-terminus of Cx43 in human embryonic kidney epithelial (293T) cells (Fig. 2). Several variants of Cx43 with amino acid deletions were generated, and the interaction of the Cx43 variants with CCN3 was analyzed using FRET analysis. A 3D model of the CCN3/Cx43 interaction complex was constructed, and through co-immunoprecipitation, CCN3 was determined to specifically bind to the C-terminus of Cx43 in the 257–382 aa region. The ability of Cx43 to reduce the growth of 293T cells was necessitated by its binding with CCN3, leading to an increase in endogenous CCN3. The signaling cascade after this binding to regulate proliferation properties remains unknown. Because the truncated Cx43 variants were still able to form functional channels, it is unlikely that GJIC is responsible for the effects on proliferation. Thus, the reduction in cell proliferation is caused by the direct interaction of CCN3 with the C-terminus of Cx43, leading to an increase in CCN3 expression. Johnstone et al. [39] studied the effects of Cx43 expression levels on the regulation of cell cycle in HeLa cells transfected with Cx43 and primary human foreskin fibroblasts (HFF), which express Cx43 predominantly. 18-α-Glycyrrhetinic acid (GA) was used to reduce GJIC but not affect Cx43 expression, and sodium butyrate (NaBu) or anti-arrhythmic peptide (AAP10) were used to enhance Cx43 expression in the cells. Using time-lapse microscopy, it was discovered that the increase in Cx43 expression levels delayed mitotic durations, which corresponded with an accumulation of cells in the G1 phase, leading to a reduction in cell proliferation. This was linked to the increased expression of p21waf1/cip1, which is a cell cycle inhibitor. These effects were independent of Cx43 channel function, which was determined by microinjection with Alexa 594 dye. Overall, it is suggested that the upregulation of Cx43 expression delays the rate of cell cycle traverse through the delay of G1, thereby increasing time between mitotic cycles. The significant influence of connexins on the cell cycle and signaling regulation of renal, skin, and neurological cancers is established by these aforementioned studies.
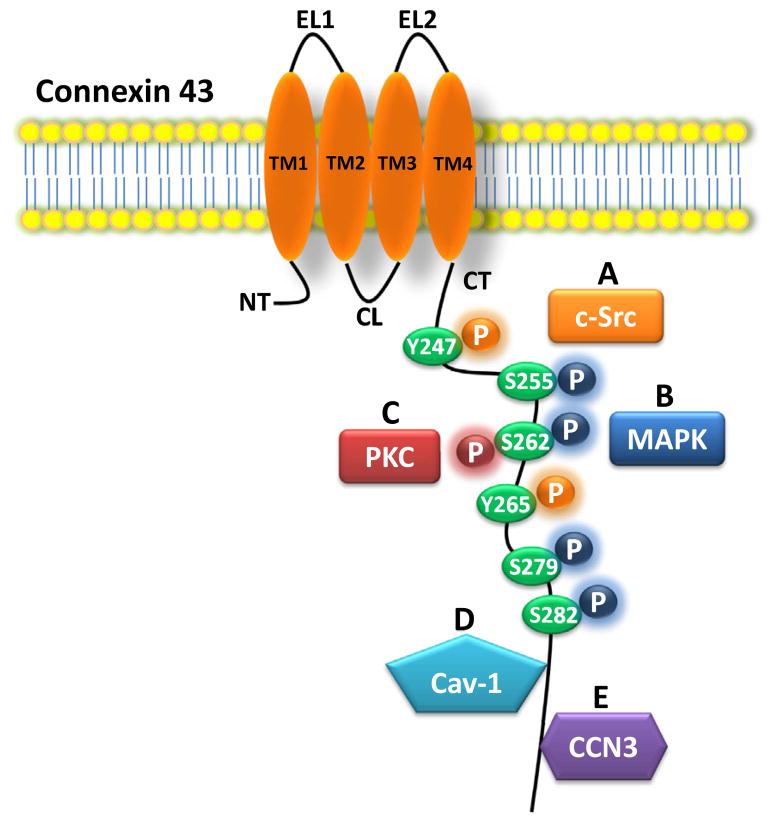
Interaction between the connexin 43 C-terminal tail and signaling factors. The C-terminal tail of Cx43 interacts with many signaling factors and regulators of cancer behavior. Phosphorylation of tyrosine 247 and 265 of the C-terminal tail inhibits c-Src activity and decreases proliferation of glioma cells [35,36] (A). Phosphorylation of serines 255, 262, 279, and 282 of the C-terminal tail by MAPK increases proliferation of vascular smooth muscle cells [52] (B). Phosphorylation of serine 262 of the CT by PKC inhibits DNA synthesis in HEK293 cells [41] and leads to cardiac ischemic injury resistance [48] (C). Interaction of the CT with Cav-1 reduces human keratinocyte skin tumor transformation and invasion [34] (D). Interaction of the CT amino acids 257–382 with CCN3 decreases growth of 293T human embryonic kidney epithelial cells [38] (E).
4. Gap junction-independent functions of connexins on nerve cell growth, migration, apoptosis and development
Many connexins also influence the behavior of cells in neurogenesis. Cx32 is suggested to play a role in Neuregulin-1 (Nrg1)-mediated proliferation which is not mediated by coupling [40]. The expression of Cx32 is upregulated in primary cultures of purified Schwann cells (SCs) from newborn mouse sciatic nerve by Glial growth factor 2 (GGF2), an isoform of Nrg1. However, SCs from Cx32 knockout mice have a smaller mitogenic response to GGF2. Additionally, there is no increase in electrical coupling between SCs following exposure to GGF2. Cx43 is known to influence the migration of cells in the brain. For example, Cx43 plays an important role in astrocytic wound closure and proliferation in response to injury [41]. First, Cx43 expression was downregulated by transfecting primary astrocyte cultures from cerebral cortices of Sprague Dawley rats with a custom plasmid-generating microRNA directed against Cx43 mRNA. The response of astrocytes to wound healing was analyzed through a modified scratch wound assay, where cells were fixed and stained for Cx43 and GFAP at appropriate times. The GJIC role was eliminated by using carbenoxolone, a gap junction and hemichannel blocker, as analyzed by FRAP with a calcein green-AM dye. As such, the wound closure rate was not affected by the addition of carbenoxolone. This data implies the influence of Cx43 in astrocyte response to injury that is separate from the intercellular communication from gap junctions or release of molecules from hemichannels. Cx43 is also shown to control the directional migration and polarized cell movement of cardiac neural crest cells (CNCs) [42]. CNCs were isolated from Cx43 knockout mice and assessed for their polarity and migration. The Cx43 knockout CNCs exhibited decreased adhesion as well as directionality. Gap junction communication was assessed using dye injections with carboxyfluorescein, and there were no associations found between the level of GJIC in these cells and changes in motility or directionality. This suggests that Cx43 affects the migration of CNCs through its signaling functions with other proteins that regulate the cell motile behavior. Both Cx43 and Cx26 are able to influence the migration of newborn neurons to the cortical plate in a fashion that does not depend on its channel functions [43]. The connexins interact with the cellular cytoskeleton to promote adhesion with the cortical cells, which enables migration. Both Cx26 and Cx43 were found to be expressed in migrating neurons and along radial fibers. The authors introduced short hairpin RNA (shRNA) constructs to knock down Cx26 and Cx43 in the developing rat brain. The mutations significantly disrupted the neuronal migration and development of the neocortex. Rescue experiments were performed in order to differentiate the exact role of connexins in neuronal migration. To determine whether functional channels are crucial to rescue the migration defect, dominant-negative mutations were introduced to the tyrosine in the third transmembrane domain of Cx26 and Cx43. These mutants are able to make adhesions but form nonfunctional channels, and they rescued the migration of cells to the cortical plate, suggesting that the connexins’ channel function does not contribute to their role in neuronal migration. Giardina et al. [44] demonstrate the influence of Cx43 on resistance against H2O2-induced apoptosis in both tumorigenic and non-tumorigenic neuronal cells in a GJIC-independent fashion. They further showed that the glioma cell death was blocked through the inhibition of caspase 3 activation. In primary astrocytes, siRNA knockdown of Cx43 enhanced the toxicity of H2O2. Thus, the protective effect of Cx43 against apoptosis is not solely found in the C6 glioma cell line, but in primary astrocytes as well. It was also shown that Cx43 protein interacts directly with the ASK1 protein, an upstream activator of JNK, from co-immunoprecipitation experiments. This is not unheard of, since Cx43 is known to interact with other protein kinases, such as c-Src, PKC, and ERK1/2 [10]. Therefore, the mechanism behind Cx43 and H2O2-induced apoptosis could possibly be attributed to the involvement of Cx43 as a signaling molecule interacting with kinases in the ASK1 pathway. Overall, these data show that connexins are involved in the directional migration of astrocytes and neurons as well cardiac neural crest cells, supporting their essential role in development.
5. Other GJIC-independent functions of connexins
Connexins have been implicated in influencing the growth, migration, and apoptosis of various cell types involved in normal differentiation and development [9,45]. Many of these studies emphasize the channel roles of connexins on cell behavior. Dang et al. [46] show how Cx43 inhibits DNA synthesis in Human Embryonic Kidney 293 (HEK293) cells through the phosphorylation of its serine (S) 262 residue in response to protein kinase C (PKC) stimulation. Because the non-channel-forming C-terminal tail of Cx43 (Cx43CT) is able to inhibit DNA synthesis through S262 regulation, the Cx43-mediated growth inhibition is shown to be independent of GJIC (Fig. 2).
Connexins have been shown to influence other organs, including the heart and lens. There are several previous studies demonstrating the involvement of connexins in blood vessel and cardiomyocyte generation, differentiation, and homeostasis [7]. Earlier studies identified the function of connexins in cardioprotection through its gap junction or hemichannel communication [47]. In a more recent study, Srisakuldee et al. [48] revealed a cardioprotective role of phosphorylated Cx43 protein. Cx43 phosphorylated at S262 and S368 represents a state of increased resistance to cardiac ischemic injury, and this is upregulated by ischemic preconditioning (PC), where isolated hearts were subjected to short episodes of ischemia followed by perfusion with oxygen, or by FGF2 treatment. These processes are shown to be dependent on protein kinase C (PKC) activity (Fig. 2). A previous study by Li and colleagues [49] showed that GJIC is not essential for ischemic PC. Thus, Cx43 is likely to mediate cardioprotection through FGF2 or ischemic PC and PKC signaling in a non-GJIC manner. It was previously shown that TGF-β signaling and blood vessel cell differentiation are mediated by Cx43 [50]. Dai et al. [51] examined the involvement of Cx43 in terminally differentiated cardiomyocytes. In this newer study, cardiomyocytes expressing endogenous Cx43 were examined, and Cx43 was shown to be involved in the regulation of TGF-β signaling through microtubule-regulated Smad signaling. Cx43 was revealed to compete with Smad2/3 for binding to β-tubulin, and its interaction with Smad2/3 was found at the microtubule level. Through the luciferase-reporter system, Cx43 was found to positively regulate TGF-β signaling. In brief, Cx43 interacts with TGF-β and regulates TGF-β/Smad signaling by triggering the release of Smad2/3 and increasing the phosphorylation of Smad2. Johnstone et al. [52] demonstrated that MAPK phosphorylates Cx43 and this causes it to interact with cyclin E in vascular smooth muscle cells (VSMC), leading to effects on proliferation (Fig. 2). Previously, they showed that Cx43 becomes phosphorylated at its MAPK serine after atherogenic stimuli, which is associated with VSMC proliferation [53].
A plethora of studies have explored the role of connexins in lens development, and mutations of these connexins have been reported to lead to human congenital cataracts [54,55]. It was previously reported that the overexpression of Cx50 stimulates lens cell differentiation [56]. In an update, Banks et al. [57] demonstrated the important role of the C-terminus of Cx50 as a key player in lens epithelial-fiber cell differentiation. Mutants of Cx50 which do not form function gap junction channels but retain the normal expression and integrity of the Cx50 protein preserved the ability to promote lens epithelial-fiber cell differentiation. Additionally, the important involvement of the Cx50 C-terminal domain in lens epithelial-fiber cell differentiation was demonstrated by developing C-terminus chimeric constructs in which the Cx50 and Cx46 C-termini were exchanged. As such, the Cx46 construct containing the Cx50 C-terminus was able to stimulate lens differentiation in a fashion similar to wild type Cx50. Thus, the authors showed for the first time that the C-terminal of Cx50 has a crucial role in lens epithelial-fiber cell differentiation independently of GJIC. To further investigate this, Shi et al. [58] established the structure of the Cx50 C-terminal domain by molecular modeling, which revealed four α-helices and a V362 residue in the middle of the 3rd helix which is functionally involved in lens epithelial-fiber differentiation and maintaining the α-helical structure. It was shown experimentally that the stimulatory effect of Cx50 on lens fiber differentiation is lessened by deletion of the PEST motif at the C-terminus of Cx50 and a mutation of the valine 362 residue. Additionally, mutation of V362 to charged residues disrupted the α-helical structure. Altogether, these experiments reinforce the significant GJIC-independent role of Cx50 in lens cell differentiation.
6. Conclusion
It is becoming more apparent that connexins serve more purposes than as mere channel proteins. They have long been known as the components of gap junction, allowing the transfer of ions and small signaling molecules between adjoining cells [3]. In more recent years, its role in hemichannel formation has also been identified [1,4]. However, its non-channel functions, independent of both gap junctions and hemichannels, are noteworthy in many physiological processes, where the connexins proteins themselves can directly interact with signaling molecules to affect cell behavior. Likewise, this reveals the complexity of its alterations and provides many implications into its effects on a wide range of disease types. Because of its many known roles in the cell, it is not surprising that there exists such a wide variety of different types of connexins. The studies we have presented reflect the importance of the connexin proteins in mediating pathways in cancer as well as the development of organs such as the brain, the lens, and the heart. Connexins have been shown to encompass multiple, sometimes contradictory, functions in cancer development and disease progression [59]. There still remains a need for future studies to examine the molecular mechanisms behind the channel-independent role of connexins in tumor growth, migration, and cell signaling, which will aid in the understanding of its many purposes in cell physiology and pathology, and provide strategies for the treatment of diseases.
Acknowledgments
The work was supported by National Institutes of Health Grant EY012085 and Welch Foundation Grant AQ-1507 to J.X.J.
References
Full text links
Read article at publisher's site: https://doi.org/10.1016/j.febslet.2014.01.001
Read article for free, from open access legal sources, via Unpaywall:
https://europepmc.org/articles/pmc4122521?pdf=render
Citations & impact
Impact metrics
Citations of article over time
Alternative metrics

Discover the attention surrounding your research
https://www.altmetric.com/details/42929858
Article citations
The Gap Junction Inhibitor Octanol Decreases Proliferation and Increases Glial Differentiation of Postnatal Neural Progenitor Cells.
Int J Mol Sci, 25(12):6288, 07 Jun 2024
Cited by: 0 articles | PMID: 38927995
Overexpression of Connexin 40 in the Vascular Endothelial Cells of Placenta with Acute Chorioamnionitis.
Diagnostics (Basel), 14(8):811, 12 Apr 2024
Cited by: 0 articles | PMID: 38667457 | PMCID: PMC11048802
The Keratinocyte in the Picture Cutaneous Melanoma Microenvironment.
Cancers (Basel), 16(5):913, 23 Feb 2024
Cited by: 1 article | PMID: 38473275 | PMCID: PMC10930874
Review Free full text in Europe PMC
Gap Junctions or Hemichannel-Dependent and Independent Roles of Connexins in Fibrosis, Epithelial-Mesenchymal Transitions, and Wound Healing.
Biomolecules, 13(12):1796, 14 Dec 2023
Cited by: 1 article | PMID: 38136665 | PMCID: PMC10742173
Review Free full text in Europe PMC
Interactive mechanism between connexin43 and Cd-induced autophagic flux blockage and gap junctional intercellular communication dysfunction in rat hepatocytes.
Heliyon, 9(10):e21052, 14 Oct 2023
Cited by: 0 articles | PMID: 37876489 | PMCID: PMC10590978
Go to all (111) article citations
Similar Articles
To arrive at the top five similar articles we use a word-weighted algorithm to compare words from the Title and Abstract of each citation.
Gap junction- and hemichannel-independent actions of connexins.
Biochim Biophys Acta, 1711(2):208-214, 22 Oct 2004
Cited by: 106 articles | PMID: 15955305 | PMCID: PMC1831832
Review Free full text in Europe PMC
Emerging issues of connexin channels: biophysics fills the gap.
Q Rev Biophys, 34(3):325-472, 01 Aug 2001
Cited by: 451 articles | PMID: 11838236
Review
Role of connexin-based gap junction channels and hemichannels in ischemia-induced cell death in nervous tissue.
Brain Res Brain Res Rev, 47(1-3):290-303, 01 Dec 2004
Cited by: 164 articles | PMID: 15572178 | PMCID: PMC3651737
Review Free full text in Europe PMC
Regulation of Connexin Gap Junctions and Hemichannels by Calcium and Calcium Binding Protein Calmodulin.
Int J Mol Sci, 21(21):E8194, 02 Nov 2020
Cited by: 13 articles | PMID: 33147690 | PMCID: PMC7663298
Review Free full text in Europe PMC
Funding
Funders who supported this work.
NEI NIH HHS (3)
Grant ID: R01 EY012085
Grant ID: R29 EY012085
Grant ID: EY012085
National Institutes of Health (1)
Grant ID: EY012085
Welch Foundation (1)
Grant ID: AQ-1507