Abstract
Introduction
Autoantibody-induced complement activation, which causes disruption of the postsynaptic membrane, is recognized as a key pathogenic factor in myasthenia gravis (MG). Therefore, specific targeting of complement inhibitors to the site of complement activation is a potential therapeutic strategy for treatment of MG.Methods
We assessed expression of single-chain antibody fragment-decay accelerating factor (scFv-DAF), comprising a single-chain fragment scFv1956 based on the rat complement inhibitor DAF in prokaryotic systems, and studied its inhibitory effect on complement deposition in vitro.Results
The recombinant conjugate scFv-DAF completely retained the wild-type binding activity of scFv1956 to AChR and inhibited complement activation of DAF in vitro.Conclusions
We found that scFv-DAF could bind specifically to TE671 cells, and it is significantly more potent at inhibiting complement deposition than the untargeted parent molecule DAF. scFv-DAF may be a candidate for in vivo protection of the AChR in MG.Free full text

PROTECTIVE EFFECT OF ScFv-DAF FUSION PROTEIN ON THE COMPLEMENT ATTACK TO ACETYLCHOLINE RECEPTOR: A POSSIBLE OPTION FOR TREATMENT OF MYASTHENIA GRAVIS
Abstract
Introduction
Autoantibody-induced complement activation, which causes disruption of the postsynaptic membrane, is recognized as a key pathogenic factor in myasthenia gravis (MG). Therefore, specific targeting of complement inhibitors to the site of complement activation is a potential therapeutic strategy for treatment of MG.
Methods
We assessed expression of single-chain antibody fragment–decay accelerating factor (scFv-DAF), comprising a single-chain fragment scFv1956 based on the rat complement inhibitor DAF in prokaryotic systems, and studied its inhibitory effect on complement deposition in vitro.
Results
The recombinant conjugate scFv-DAF completely retained the wild-type binding activity of scFv1956 to AChR and inhibited complement activation of DAF in vitro.
Conclusions
We found that scFv-DAF could bind specifically to TE671 cells, and it is significantly more potent at inhibiting complement deposition than the untargeted parent molecule DAF. scFv-DAF may be a candidate for in vivo protection of the AChR in MG.
Myasthenia gravis (MG) is an autoimmune disease in which antibodies directed to the nicotinic acetylcholine receptor (nAChR) at the postsynaptic neuromuscular junction (NMJ) cause neuromuscular transmission defects, culminating in weakness and fatigue of skeletal muscles.1-3 Although the bivalent autoantibody that was assumed to be the main pathogenic factor can modulate AChRs by cross-linking and accelerating endocytosis and degradation, as well as directly blocking AChR function, overwhelming evidence has demonstrated that it is the activation of complement through successive stimulation of C3 and C5 convertase that finally results in focal lysis of the postsynaptic membrane.4-6 Complement deposition has been observed at myasthenic NMJs of human subjects with MG and in animals with experimental autoimmune myasthenia gravis (EAMG).7,8 Complement seems to be a critical mediator of anti-AChR antibody pathogenicity in MG. Complement-inhibitor therapy has been shown to be effective in EAMG. The severity of EAMG can be alleviated by cobra venom factor,4 a monoclonal antibody (MAb) against C5,9 or a soluble form of complement receptor 1.10
The main regulatory membrane inhibitors of complement activation located on cell surfaces include complement receptor 1 (CR1; CD35), decay-accelerating factor (DAF; CD55), and membrane cofactor protein (MCP; CD46). These membrane regulators shield cells from activation of autologous complement on their surfaces and subsequent complement-mediated destruction.11 Among these proteins, DAF seems to be the most important in protection of muscle against complement-mediated injury in EAMG.12 A previous study demonstrated that, compared with wild-type littermates, Daf1-deficient animals were highly sensitive to EAMG induction and underwent more severe complement-mediated muscle damage.13 Therefore, administration of DAF may be a potential therapeutic strategy in treating MG.
Several approaches have been shown to facilitate attachment of complement-regulatory agents to a cellular surface by a targeting vehicle, which would enhance the efficacy of complement regulators compared with the unattached parent agents.14-16 Zhang and colleagues used a recombinant chimera comprising CD59 or DAF joined to an anti-5-dimethylaminonaphthalene-1-sulfonyl (anti-dansyl) antibody fragment, a targeting vehicle, which successfully targeted both CD59 and DAF to the hapten dansyl on the surface of Chinese hamster ovary (CHO) cells.17,18 In addition, using the same approach, human DAF has been tagged to a clinically relevant target cell type, a red blood cell (RBC), with an scFv specific for TER-119, which is an RBC-restricted surface antigen of the mouse.19 One study demonstrated that the effectiveness of soluble DAF at inhibiting complement-mediated lysis can be significantly enhanced when it is targeted to cell membranes via a glycophosphatidylinositol (GPI) anchor.14 However, DAF molecules containing their targeting vehicle are targeted at a non-specific cell type for certain diseases. Therefore, there remain some unresolved questions related to the clinical use of systemic anti-complement agents. The effect of systemic complement inhibition on the capacity to prevent host infections is not yet clear, as reflected in immunocompromised MG patients. The capacity for complement inhibition and safety of anti-complement agents may be enhanced by the presence of DAF that targets the molecule to pathologic sites where complement activation occurs in MG. Moreover, specific targeting may allow a much lower effective serum concentration of agents.
The key challenge of this study is how to specifically deliver DAF to the pathologic target sites of MG. As shown by previous research, univalent antibody fragments, such as fragment antigen-binding (Fab) or scFv, are not pathogenic; in contrast, such fragments derived from anti–main immunogenic region (anti-MIR) MAbs can shield the MIR from the binding of pathogenically intact antibodies and MG autoantibodies without affecting ion channel function.20-22 The previously constructed univalent antibody fragment scFv195, which is derived from anti-MIR MAb195, has been shown to be a good candidate therapeutic molecule.23 The use of the scFv1956 fragment in this study is expected to allow the fusion protein to specifically target cells or tissues with the MIR of nAChR. In this study, we assessed the feasibility of targeting the novel hybrid molecule scFv-DAF to the site of complement activation triggered by pathologic antibodies to the AChR.
METHODS
Construction of Recombinant Plasmid
cDNA clones of the scFv1956 in vector pHENl were kindly provided by Dr. Socrates J. Tzartos (Hellenic Pasteur Institute, Greece).23,24 The DNA fragment encoding short consensus repeats (SCRs)1–4 of rat DAF was amplified by polymerase chain reaction (PCR), using rat DAF cDNA as the template (NCBI Accession No. AB026902).25 The cDNA of scFv1956 and SCRs1–4 of rat DAF were joined by a 45-base spacer which codes for the flexible 15-amino-acid linker (Gly4Ser1)3, as previously described.26 Based on the nucleotide sequences of scFv1956 and rat DAF, four oligonucleotide primers were designed to amplify the cDNA fragments of the fusion protein (Table 1).
Table 1
Four primers for constructing the fusion protein.
Primer sequence (5′ to 3′) | Underline bases | |
---|---|---|
P1 | TTTCATATGCAGGTCCAATTTGTAGAG | NdeI |
P2 | ATCTCAGAAGAGGATCTGAATTCAGGTGGAGGCGGTTCAG![]() | scFv1956 bases for overlap |
P3 | GTCTGGAGGTGGGCCGCAGTCCGATCCGCCACCGCCAGAGC![]() | Rat DAF bases for overlap |
P4 | TTTTGGATCCTTATCTATGCACTTGG GTGGT | BamHI |
The specific primers P1 and P3 were used to amplify the scFv1956–linker sequence, and the specific primers P2 and P4 were used to amplify the linker–DAF sequence. Amplification of cDNA encoding scFv1956 and DAF was performed using DNA polymerase (Primer STAR HS; Takara, Japan). Purified scFv1956–linker and linker–DAF fragments were mixed at an approximate 1:1 molar ratio and used as the template, with the addition of P1 and P4 primers, and these two fragments subsequently overlapped.27
The complete fusion fragment, scFv-DAF, was digested using the endonucleases NdeI and BamHI (Takara, Japan), and cloned into the corresponding sites of the prokaryotic expression vector plasmid pET16b (Novagen). The reconstructed plasmid was verified by restriction enzyme analysis and direct sequencing. A schematic of the plasmid construction is shown in Figure 1.
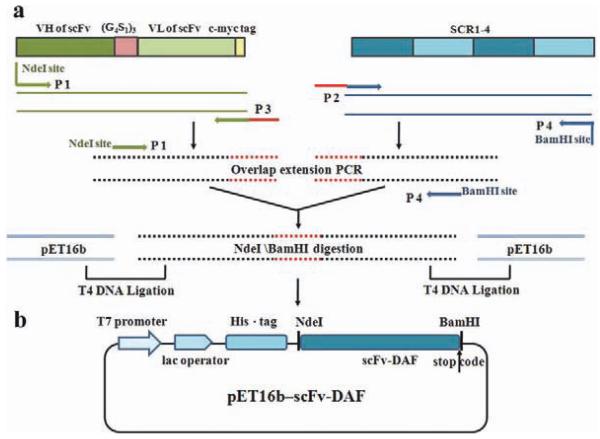
Construction of the expression vector pET16b–scFv-DAF. (a) Schematic representation of strategy for reconstruction of scFv and DAF chimeric gene. (b) Detailed sequence features of the scFv-DAF fusion protein. [Color figure can be viewed in the online issue, which is available at wileyonlinelibrary.com.]
Expression, Purification, and Renaturation of Recombinant scFv-DAF
BL21 (DE3) pLyss (Invitrogen) bacteria transformed with the reconstructed plasmid pET16b were grown at 37°C in 2× YT medium containing 100 μg/ml ampicillin and 50 μg/ml chloramphenicol. Cells were induced with 1 mM isopropyl-β-d-thiogalactoside (IPTG) for 4 h according to the plasmid manufacturer’s instructions (Novagen). The fusion protein was expressed as inclusion bodies and purified using chelating high-performance columns (Hitrap; GE Healthcare), according to the manufacturer’s protocol. The protein elution was analyzed by 12% sodium dodecylsulfate–polyacrylamide gel electrophoresis (SDS-PAGE), and protein concentration was calculated using the Bradford assay.28 Purified inclusion bodies were then refolded using the optimized urea gradient dialysis method as described previously.29
SDS-PAGE and Western Blot
The refolded fusion protein scFv-DAF was run on 12% SDS-PAGE gels, and separated protein bands were visualized by staining with Coomassie Blue R-250 or transferred to polyvinylidene fluoride membranes (Millipore) for Western blot analysis. The membranes were blocked with 5% (w/v) nonfat dried milk in phosphate-buffered saline (PBS)–Tween 20 (0.05%) for 1 h at room temperature and incubated with primary antibody at 4 °C overnight. scFv1956 and DAF were probed with the anti–c-myc tag MAb9E10 (Santa Cruz) and anti-DAF MAb at 1:1000 dilution. Membranes were washed with PBS–Tween 20 (0.05%) and then incubated with secondary antibody IRDye 800CW-conjugated goat anti-mouse IgG (1:8000 dilution) in PBS 5% (w/v) nonfat dried milk for 2 h. After washing, the bands on the membrane were visualized and photographed using an Odyssey image system (Li-Cor).
Fusion Protein scFv-DAF Binding Capacity Assays
The binding capacity of scFv-DAF to the human AChR was assessed using an enzyme-linked immunoassay (ELISA) system.30 The N-terminal extracellular domain (amino acids 1–210) of the α-subunit of AChR, named hAChRα1–210, contains the MIR that can be targeted by most pathologic autoantibodies and scFv1956.20,23 Wells of microtiter plates were coated with diluted hAChRμ1–210 at the optimal coating concentration (2 μg/ml) and incubated overnight at 4 °C. After washing three times with PBS–Tween 20 (0.05%), the plates were blocked with 5% nonfat dry milk in PBS and incubated at 37 °C for 1 h. After the washing step, different dilutions of the refolded fusion proteins scFv-DAF and scFv1956 were added to the plates and incubated for 1 h at 37 °C. After a triple washing cycle, anti-c-myc tag MAb9E10 (Santa Cruz), at a 1:5000 dilution, was added, and plates were incubated for 1 h at 37 °C. After an additional washing step, horseradish peroxidase (HRP)-conjugated rabbit anti-mouse IgG antibody (ZSBiO, China) (1:8000) was added, and the plates were incubated for 1 h at 37 °C. After the final washing step, the reaction was visualized using 1,2-phenylenediamine dihydrochloride (OPD; GenView) as substrate. The color developed after 20 min was measured at 495 nm using a microtiter plate reader (Bio-Tek Synergy HT).
Hemolytic Assay
Classical pathway hemolysis assays were performed as described previously31 to compare the complement-inhibitory activity of scFv-DAF with that of unmodified DAF. In brief, anti-body-coated sheep erythrocytes were used as target cells and incubated with different concentrations of test protein and 3% guinea pig serum (as a source of complement) at 37 °C for 1 h. The suspension was then centrifuged, and the supernatant was isolated. The serum, proteins, and cells used in this experiment were diluted in gelatin veronal buffer (GVB). Erythrocyte lysis was assessed by measuring hemoglobin release, as indicated by the absorbance value of the supernatant at 410 nm. Inhibition of hemolysis was calculated as described previously.32
In Vitro Complement Deposition Assays on TE671 Cells
The human rhabdomyosarcoma cell line TE671 [American Type Culture Collection (ATCC)], which expresses AChR on its surface,33 was used as an in vitro complement attack model. Because they are sarcoma cells, TE671 cells express complement regulator proteins on the cell surface.34 Therefore, monoclonal antibodies against DAF were used to block the functional effects of these complement regulators (incubation for 2 h at 37 °C) prior to the initial serum sensitization.35,36 The rat hybridoma Tib-175, producing the anti-AChR MAb35, was obtained from the ATCC. MAb35, which is a highly pathogenic rat anti-AChR monoclonal antibody specific for the MIR, was purified as described previously.37,38
TE671 cells were cultured in Dulbecco modified Eagle medium (DMEM; Gibco) supplemented with 10% heat-inactivated fetal bovine serum (FBS; Gibco) and antibiotics (penicillin 100 U/ml, streptomycin 100 μg/ml, both from Gibco) in a humidified atmosphere of 5% CO2 at 37 °C.39 Confluent TE671 cells were sensitized with MAb35 at 1:20 dilution for 40 min at 37° and then incubated with scFv-DAF (100 nM), scFv1956 (100 nM), and DAF (100 nM) at 37 °C for 45 min. After incubation (45 min, 37 °C), cells were washed to remove unbound scFv-DAF, scFv1956, and DAF. Freshly frozen guinea pig serum was used as a source of complement; it was thawed gradually and incubated with TE671 cells on ice for 45 min to remove natural antibodies and reduce subsequent non-specific complement activation. The pre-adsorbed guinea pig serum was applied to the sensitized TE671 cells at a 1:20 dilution and incubated for 40 min at 37°. The cells were then fixed in 4% formaldehyde and stained with fluorescein isothiocyanate (FITC)-conjugated anti-C3 antibody (ViroStat), diluted with eosin staining solution as contrast stain at 4 °C overnight to prevent the loss of C3 fragments from the cell surface.
TE671 cells, after exposure to a complement source as described, were then washed three times in PBS and resuspended in PBS containing a working dilution of FITC-conjugated goat anti-mouse C3 antibody and incubated for 30 min on ice. PBS was used as the negative control. A minimum of 20,000 gated event samples were collected on a FACSCalibur (Elite ESP; Beckman Coulter) and analyzed using CellQuest software.
Statistical Analysis
To determine the significance of the observed results, the Student t-test was used for testing the binding capacity of scFv-DAF to human AChR and the complement-inhibitory activity of scFv-DAF. Statistical significance was defined as P < 0.05.
RESULTS
Construction, Expression, and Purification of scFv-DAF Fusion Protein
The scFv-DAF gene fragment containing the flexible linker sequence and restriction sites (1578 bp) was amplified and cloned into the prokaryotic expression vector pET16b. Restriction enzyme analysis and subsequent sequencing confirmed that the reconstructed plasmid included scFv-DAF as expected, with no extra mutations introduced by PCR. The sequence-verified constructed plasmid, pET16b-scFv-DAF (see Fig. 1) was then transformed into BL21 (DE3) pLyss cells.
Samples from the pre- and post-induction purification and refolding of scFv-DAF were separated by 12% SDS-PAGE and stained with Coomassie brilliant blue (Fig. 2). An additional band at 61 kDa was present in the post-induction sample (lane 2 in Fig. 2, black arrow), indicating expression of scFv-DAF. The yield of the purified fusion protein was estimated at 20 mg/L of bacterial culture. Western blot analysis revealed a protein of 61 kDa, consistent with the predicted molecular mass of scFv-DAF under reducing conditions (lanes 2 and 5 showing white arrows in Fig. 3). Due to the attached c-myc tag peptide behind scFv, the purified scFv-DAF was detected by the anti–c-myc tag MAb9E10 and the monoclonal anti-DAF antibody (Fig. 3). ScFv1956 and DAF were used as controls.
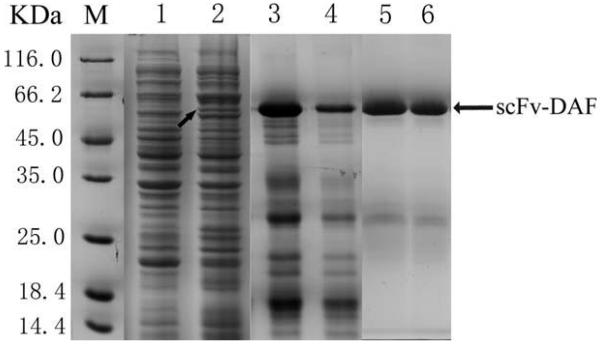
Expression and purification of fusion protein. 1—pET16b–scFv-DAF/BL21 (DE3) plyss before induction; 2—pET16b–scFv-DAF induced by 1 mM IPTG for 4 h; 3 and 4—eluted peak of fusion protein from Hitrap chelating HP column; 5 and 6—pET16b–scFv-DAF refolded with the optimized urea gradient dialysis method. M, low molecular protein markers. The black arrow on lane 2 indicates the additional band at 61 kDa.
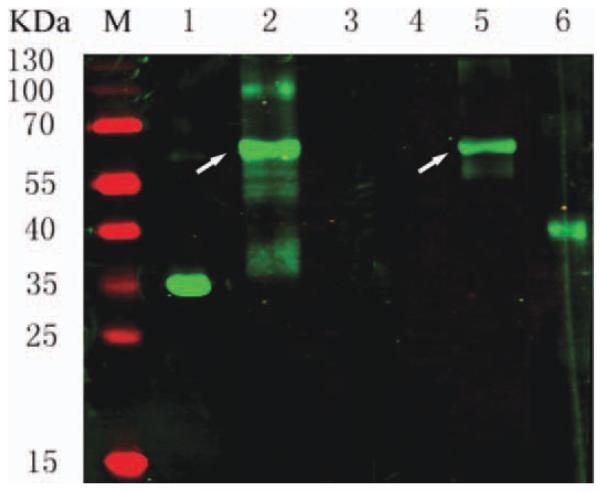
Western blot analysis of fusion protein. Lanes 1–3 were detected with an anti–c-myc tag MAb9E10; Lanes 4–6 were probed with an anti-DAF MAb. Controls included scFv1956 (lanes 1 and 4) and DAF (lanes 3 and 6). The white arrows on lanes 2 and 5 indicate the fusion protein scFv-DAF. [Color figure can be viewed in the online issue, which is available at wileyonlinelibrary.com.]
Binding Characteristics of scFv-DAF to AChR
The binding ability of scFv-DAF to human AChR was further examined using an ELISA-based assay. As shown in Figure 4, hAChRα1–210 was selectively captured in ELISA plate wells by both scFv1956 and scFv-DAF. Compared with scFv-DAF, scFv1956 has a slightly higher binding affinity to hAChRα1–210, especially when the protein concentration exceeded 800 nM. Similar results were obtained when different concentrations of hAChRα1–210 were immobilized on plastic plates (data not shown). ELISA assays confirmed that the renatured scFv-DAF can bind specifically to hAChRα1–210 and that the construct can be recognized by anti-DAF MAb (data not shown). These results indicate that scFv-DAF attained a native structure of scFv1956. Together, these results show that addition of the complement regulator DAF has little effect on the interaction between scFv-DAF and hAChRα1–210.
In Vitro Complement-Inhibitory Activities of scFv-DAF
Hemolytic assays were performed to determine whether DAF can still inhibit complement activation after N-terminal modification with scFv1956. The concentration of rat serum used in these experiments (3%) resulted in 100% lysis of unprotected erythrocytes (with only added GVB), whereas 0.5 M ethylene-diamine tetraacetic acid (EDTA) provided antibody-sensitized sheep erythrocytes with 100% protection. Antibody-sensitized sheep erythrocytes were incubated with DAF or scFv-DAF in the presence of 3% rat serum in GVB.
The complement-inhibitory activity of scFv-DAF was found to be within the range of activity previously reported for recombinant soluble DAF (Fig. 5).14 However, compared with DAF, there was little difference in the protection of antibody-sensitized sheep erythrocytes provided by scFv-DAF at high concentrations, indicating that the scFv-targeting moiety had few adverse effects on the function of DAF.
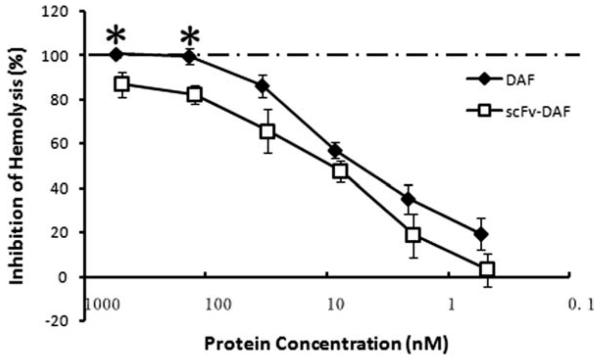
In vitro complement-regulatory function of scFv-DAF. The degree of complement-mediated hemolysis was quantified by release of hemoglobin to the supernatant and plotted as molar concentration of inhibitor present in the assay. Each data point represents the mean of three individual experiments. Error bars represent SD values. *P < 0.05.
In Vitro Complement Deposition Assays on TE671 Cells
The results just outlined indicate that scFv-DAF is capable of binding hAChRα1–210 and that it retains complement-regulatory activity in vitro. To assess the potential suitability of scFv-DAF as a candidate for in vivo protection of AChR in MG, we examined its ability to protect TE671 cells against complement-mediated lysis. To confirm this, MAb35-sensitized TE671 cells were incubated in the presence of DAF and scFv-DAF and then washed before exposure to complement. As expected, TE671 cells were protected by DAF to a limited extent from complement-mediated attack (DAF group, Fig. 6D and I). However, scFv-DAF provided TE671 cells with adequate protection from complement-mediated attack, indicating that the fusion protein had bound firmly to the cell membrane (scFv-DAF group, Fig. 6B and G). The presence of scFv-DAF resulted in an approximately 50% reduction in C3 deposition (mean relative fluorescence) compared with control, whereas scFv1956 and DAF resulted in 23% and 20% reductions, respectively, in C3 deposition (Fig. 7). Immunofluorescence and flow cytometry analysis demonstrated that scFv-DAF reduced complement-mediated attack on the TE671 surface compared with scFv1956 and DAF, likely because the targeting vehicle, scFv, can specifically bind to AChR (Figs. 6 and and77).
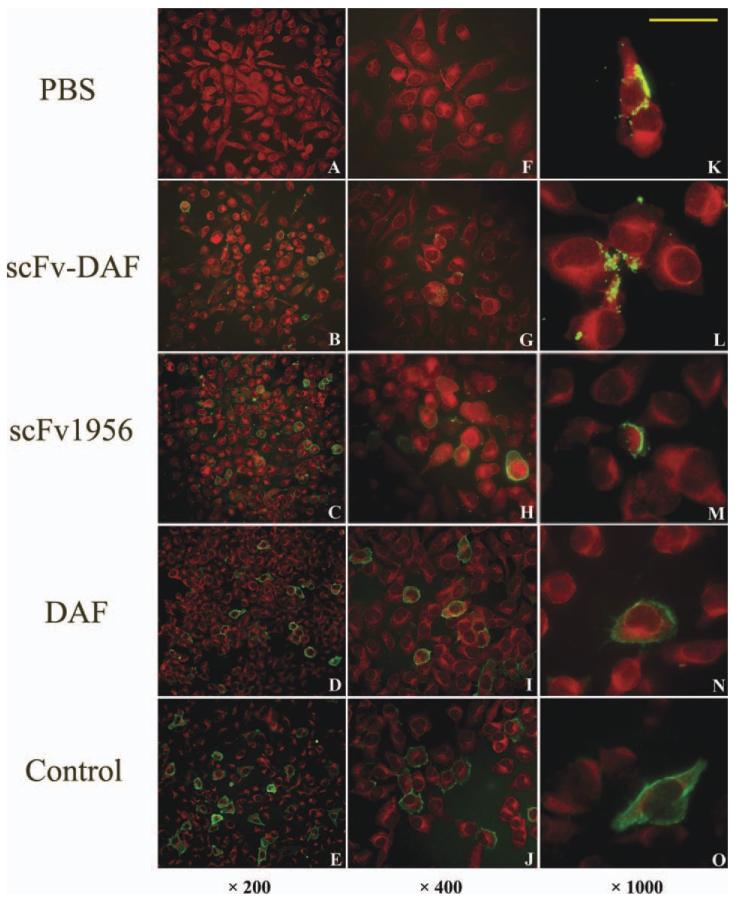
The scFv-DAF fusion protein reduces C3 deposition on TE671 cells. TE671 were stained with FITC-conjugated anti–complement 3 (anti-C3) antibody (green), and stained with eosin staining solution (red) as contrast. Negative controls were obtained by substituting MAb35 with PBS (A, F). TE671 cells treated with normal basal medium in the presence of 100 nM scFv-DAF (B, G), scFv1956 (C, H), and DAF (D, I). Positive controls were obtained in the absence of inhibitor (E, J). Representative immunofluorescence image of complement deposition on the surface of TE671cells (K–O). The staining of C3 deposition is patchy and moderate (K, L), and was observed in the presence of scFv-DAF and scFv1956. The staining of C3 deposits around the TE671 cell is orbicular (M–O), and was observed in the presence of DAF and positive control. Scale bar = 10 μm. [Color figure can be viewed in the online issue, which is available at wileyonlinelibrary.com.]
DISCUSSION
In this study we have demonstrated that the AChR-targeted fusion protein scFv-DAF selectively bound to TE671 cells was more potent at inhibiting complement deposition than the unmodified DAF protein. We found that the expression of scFv-DAF, which is based on the rat complement inhibitor DAF in prokaryotic systems, produces an efficiently folded, biologically active molecule. The recombinant conjugate, scFv-DAF, was shown to completely retain scFv1956 binding activity to AChR and the DAF inhibitory effects on complement activation in vitro.
Hemolysis assays indicated that the scFv-DAF fusion protein was an effective inhibitor of complement activation in an untargeted system, and its activity was within the range of that of recombinant soluble DAF in vitro, as reported previously14 (see Fig. 5). When incorporated on TE671 cell surfaces, the effectiveness of scFv-DAF at protecting TE671 cells from complement-mediated deposition was twofold greater than that of unmodified soluble DAF, possibly owing to its specific targeting and tight binding to the sites where complement activation occurs. It has been shown previously that the efficiency of DAF at inhibiting complement activation is greatly enhanced when DAF containing its GPI anchor is inserted into a cell membrane.14,40 However, DAF containing its GPI anchor will insert into any membrane and is not targeted to a specific cell type. The scFv-DAF construct can potentially provide protection to cell or tissue populations that are only recognized by a specific antibody, scFv1956.
It is of interest that some anti-complement activity was also observed with the unmodified DAF after the wash step, when it was expected that free inhibitors should have been removed. Prior to adding unattached soluble DAF, intrinsic DAF on the surface of TE671 cells had been pre-blocked by anti-DAF MAb. It is assumed that some of the intrinsic DAF was exposed, because anti-DAF MAb was competitively bound to unattached soluble DAF and removed by the wash assay.
To increase the effectiveness of targeted complement inhibitors of activation, the targeting vehicle should be better addressed. With regard to either orientation or distance from the site of C3 convertase formation, the inhibitory effects of cell-bound DAF on complement activation could be affected by positioning of the targeting vehicle; indeed, previous work has indicated that a small targeting vehicle is preferable to a large one.17,18 In addition, in the case of antibody targeting, as in this study, the location of the epitope relative to the cell membrane may influence the activity of a cell-targeted complement inhibitor. For this study, there are several reasons to have selected scFv1956 as a targeting vehicle that could direct DAF to the surface of the endplate. First, because the large proportion of pathogenic antibodies were directed against the MIR located at each of the two α-subunits of the AChR,20,41 scFv1956 derived from high-affinity anti-MIR MAb195 was selected for specifically binding to pathologic site AChR.23 Second, univalent single-chain Fv fragments of anti-MIR MAbs cannot themselves cross-link AChRs; therefore, their use avoids the accelerated endocytosis and degradation of AChR. Third, the conjugate scFv-DAF, containing the small targeting vehicle scFv1956, functioned more effectively than a conjugate containing a large targeting vehicle due to its closer positioning to the target cell surface. Furthermore, other factors, such as the type of linkage or spacer between the antibody domains and DAF, and the surface antigen density could also affect the activity of cell-bound scFv-DAF.
Another study showed that complement is involved in the pathogenesis of various inflammatory and autoimmune diseases, and some soluble anti-complement agents have been shown to be protective against cell and tissue injury in several animal models, including EAMG.42,10 Anti-complement therapeutic agents may be adequate for acute, short-term inhibition of complement disease. However, rapid clearance in vivo of soluble recombinant complement regulators led to the demand for sustained administration, rendering them inappropriate for long-term treatment and limiting their clinical application. Although fusion of DAF or CD59 with human immunoglobulin Fc domains markedly prolonged the half-life of the conjugates,31,43 the biological activity of both complement regulators was reduced. Although it is unknown whether long-term administration will impair the host defense function, it should be noted that the susceptibility to infection and immune complex diseases may increase. Targeted application aims to avoid these potential systemic side effects. Therefore, the development of therapeutic agents for chronic diseases such as MG is required to specifically target complement regulators to the pathologic site and achieve successive complement inhibition over a long therapeutic period. The issue of rapid clearance of anti-complement agents may be solved by specific targeting. MG is a typical chronic disease with organ-specific damage, and targeting DAF with scFv1956 to neuromuscular junctions may solve these problems.
In our study the complement-regulatory protein DAF conjugated to the targeting vehicle anti-MIR scFv1956, which has very high affinity for the human AChR, was engineered, and our results indicate that it may be a candidate for in vivo protection of the AChR in MG. However, our investigation has several limitations. We only evaluated the function of scFv-DAF fusion protein in vitro, so we do not know whether DAF can be delivered to the AChR at the NMJ and protect the endplate from complement-mediated damage in EAMG. The clearance rate of scFv-DAF from the circulation should also be observed in further studies.
In conclusion, we have provided an example of ameliorating cellular damage through site-specific complement inhibition in vitro, which may offer a new therapeutic application not only for MG, but also for other human complement-mediated diseases.
Acknowledgments
The authors thank Professor Angela Vincent (Neurosciences Group, Weatherall Institute of Molecular Medicine, Oxford) for providing the TE671 cell line for our research. This work was supported by the National Natural Science Foundation of China (30972721 and 30870841). H.J.K. was supported by the National Institutes of Health (R24 EY014837).
Abbreviations
AChR | acetylcholine receptor |
ATCC | American Type Culture Collection |
CHO | Chinese hamster ovary |
CR1 (CD35) | complement receptor 1 |
C3 | complement 3 |
DAF (CD55) | decay accelerating factor |
dansyl | 5-dimethylaminonaphthalene-1-sulfonyl |
DMEM | Dulbecco’s modified Eagle medium |
EAMG | experimental autoimmune myasthenia gravis |
ELISA | enzyme-linked immunosorbent assay |
Fab | fragment antigen-binding |
FBS | fetal bovine serum |
FITC | fluorescein isothiocyanate |
GPI | glycophosphatidylinositol |
GVB | gelatin veronal buffer |
HRP | horseradish peroxidase |
Hα1–210 | extracellular domain of human AChR α1 subunit |
IPTG | isopropyl β-d-thiogalactoside |
MAb | monoclonal antibody |
MCP (CD46) | membrane cofactor protein |
MG | myasthenia gravis |
MIR | main immunogenic region |
NMJ | neuromuscular junction |
OPD | 1,2-phenylenediamine dihydrochloride |
PVDF | polyvinylidene fluoride |
RBC | red blood cell |
scFv | single-chain Ab fragment |
SCR | short consensus repeat |
SDS-PAGE | sodium dodecylsulfate–polyacrylamide gel electrophoresis |
REFERENCES
Full text links
Read article at publisher's site: https://doi.org/10.1002/mus.23247
Read article for free, from open access legal sources, via Unpaywall:
https://europepmc.org/articles/pmc4308948?pdf=render
Citations & impact
Impact metrics
Citations of article over time
Alternative metrics

Discover the attention surrounding your research
https://www.altmetric.com/details/105049865
Smart citations by scite.ai
Explore citation contexts and check if this article has been
supported or disputed.
https://scite.ai/reports/10.1002/mus.23247
Article citations
Receptor clustering and pathogenic complement activation in myasthenia gravis depend on synergy between antibodies with multiple subunit specificities.
Acta Neuropathol, 144(5):1005-1025, 08 Sep 2022
Cited by: 16 articles | PMID: 36074148 | PMCID: PMC9547806
A Targeted Complement Inhibitor CRIg/FH Protects Against Experimental Autoimmune Myasthenia Gravis in Rats via Immune Modulation.
Front Immunol, 13:746068, 26 Jan 2022
Cited by: 3 articles | PMID: 35154091 | PMCID: PMC8825366
Halting targeted and collateral damage to red blood cells by the complement system.
Semin Immunopathol, 43(6):799-816, 30 Jun 2021
Cited by: 6 articles | PMID: 34191092 | PMCID: PMC8243056
Review Free full text in Europe PMC
Therapeutic Lessons to be Learned From the Role of Complement Regulators as Double-Edged Sword in Health and Disease.
Front Immunol, 11:578069, 10 Dec 2020
Cited by: 8 articles | PMID: 33362763 | PMCID: PMC7758290
Review Free full text in Europe PMC
Complement Inhibitor Therapy for Myasthenia Gravis.
Front Immunol, 11:917, 03 Jun 2020
Cited by: 32 articles | PMID: 32582144 | PMCID: PMC7283905
Review Free full text in Europe PMC
Go to all (10) article citations
Data
Data behind the article
This data has been text mined from the article, or deposited into data resources.
BioStudies: supplemental material and supporting data
Nucleotide Sequences
- (1 citation) ENA - AB026902
Similar Articles
To arrive at the top five similar articles we use a word-weighted algorithm to compare words from the Title and Abstract of each citation.
Targeting therapy to the neuromuscular junction: proof of concept.
Muscle Nerve, 49(5):749-756, 01 May 2014
Cited by: 17 articles | PMID: 24037951 | PMCID: PMC4296224
Targeting of functional antibody-decay-accelerating factor fusion proteins to a cell surface.
J Biol Chem, 276(29):27290-27295, 14 May 2001
Cited by: 14 articles | PMID: 11352906
Decay accelerating factor (CD55) protects neuronal cells from chemical hypoxia-induced injury.
J Neuroinflammation, 7:24, 09 Apr 2010
Cited by: 27 articles | PMID: 20380727 | PMCID: PMC2867804
Complement associated pathogenic mechanisms in myasthenia gravis.
Autoimmun Rev, 12(9):904-911, 26 Mar 2013
Cited by: 57 articles | PMID: 23537510
Review